Opt for mixed land use to generate clean energy
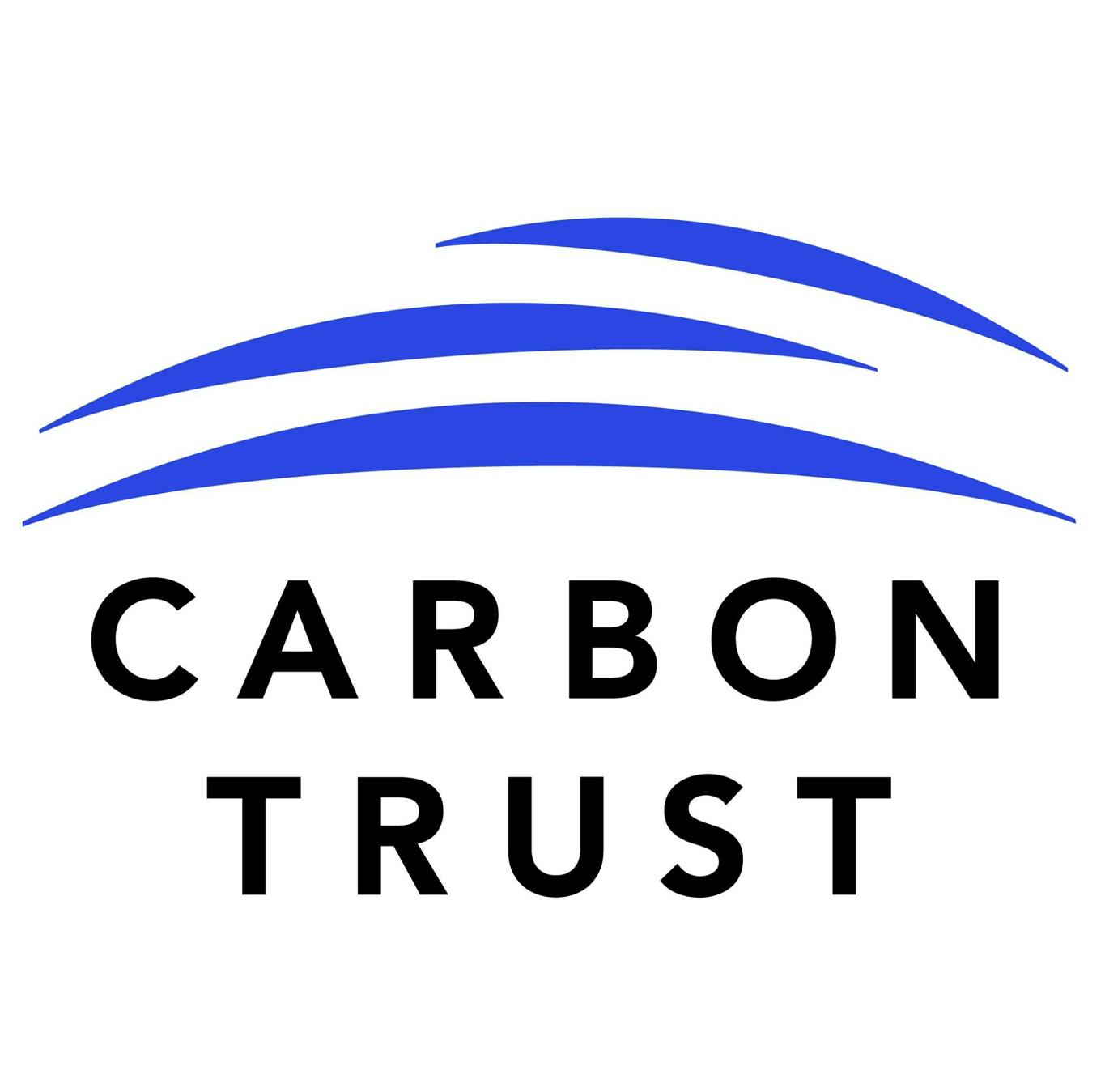
Summary
Mixed land use provides an opportunity to generate renewable energy while improving land productivity and enhancing livestock welfare
Key resources
Context
Agriculture accounts for 14% of global emissions (1) and 50% of the world’s habitable land (2). As the global population grows, the sector will need to produce 50% more food by 2050 (3), which could lead to increased emissions from production. This would exacerbate the sector’s contribution to climate change and lead to increased demand for land.
Simultaneously, expanding renewable energy capacity is essential to achieving Net Zero, and will also require vast areas of land. This has raised concerns that renewable energy will displace farming (4). Land use requirements for solar energy production vary globally between 0.5-5% of total land (5). In Germany, for example, land required for ground-mounted solar panels (also known as photovoltaics or PV) is expected to triple by 2030, and 1.7% of agricultural land will need to be converted by 2040 (6).
Both the food and energy systems must accommodate increasing demand while maintaining progress toward Net Zero. There will be land use conflict to meet demand for human habitation, agriculture, and renewable energy production without encroaching on natural wildlife. This could increase food prices and scarcity, and force one system to be prioritized at the expense of others. Studies suggest that the land with the greatest solar power potential — based on incoming sunlight, air temperature and relative humidity — is current cropland (7).
Mixed land use, a method of combining functional land uses, provides an opportunity to reconcile these competing priorities and minimize tradeoffs. Evidence suggests that mixed land use can support the twin goals of expanding renewable energy production and growing agricultural productivity, while supporting sectoral decarbonization. One study estimated that co-developing 1% of the world’s cropland with solar panels could satisfy the entire global demand for electricity (7).
Figure 1: Annual agricultural production emissions could reach 9 gigatons or more by 2050

Figure 2: Agriculture accounts for about one-quarter of global GHG emissions (~2010)

Solution
Mixed land use (or agricultural dual use) means using the same area of land for both agricultural practices and generating renewable energy. This includes agrivoltaics, where crops are grown either between or under solar panels. Agrivoltaics can be operated to support a range of agricultural practices, including a variety of crop types and livestock models. Using the same plot for both activities can increase land use efficiency by up to 186% (8).

For open agrivoltaic systems (those in open fields as opposed to greenhouses), there are two main methods:
Overhead PV, where crops are planted directly beneath solar panels
Ground-level interspace PV, which typically uses the space between ground-mounted panels for farming
Within these two main categories, every agrivoltaic system is different, depending on:
The type of solar panel used
The layout of the solar panels, including
Panel density
Row spacing
Crop placement
Overhead PV
Overhead PV is the most common method in use. Growing crops underneath solar panels increases land efficiency and helps improve crop productivity by protecting them from environmental conditions. For example, overhead panels provide more protection from sunlight than ground-mounted systems; as such, they are more compatible with shade-tolerant crops. (8).
Overhead panels are installed at least 2.1 meters above the ground (8), with periodic spacing to allow sufficient sunlight to reach the plants (10).
Ground-mounted interspace PV
Ground-mounted interspace systems are often a lower cost option and create less impact on the landscape (as no foundations need to be dug) (8). They are commonly used for integration with livestock and arable crops.
Interspace systems can also incorporate roving structures. These consist of panels mounted onto automated machinery that can move across croplands throughout the day to increase access to sunlight (10).
Both overhead and interspace systems are compatible with a range of crop types, including:
Permanent perennials (fruits, berries, viticulture)
Single-year long-term crops (arable crops, vegetables)
Permanent grassland (commercial grassland)
Permanent grassland pasture (for livestock) (8)
Types of solar panels
Selecting the right type of solar panel is key to crop productivity in both systems. There are many different types available, for instance:
Standard panels, installed at a 30⁰ angle for maximum efficiency across a broad range of applications (11) vs. bifacial vertical panels, which stand upright and can absorb light on both sides (12)
Fixed panels vs. panels that can tilt to follow sunlight (10)
Opaque (standard) translucent or transparent solar panels (12)
The requirements of the crop and land being worked on will determine what type of solar panel is most suitable for an organization. For example, semi-transparent panels minimize shade and are therefore suitable alongside crops like tomatoes and basil, which require prolonged exposure to full sunlight. Dynamic arrays, called roving arrays in ground-mounted systems and tracking arrays in overhead systems, prevent any one area from being permanently shaded.
Layout of solar panels
Figure: Examples of Agrivoltaic Layouts, A depicting overhead and B/C depicting ground-mounted interspace systems



Source: Fraunhofer (2022)
Groups of solar panels are known as arrays. Factors such as the height of arrays, the amount of space between rows of solar panels, and the total density of panels within a plot of land can significantly influence crop growth. (15) Arrays can be configured to optimize factors such as shade, light, and soil moisture. Configuring solar panels appropriately is essential for optimizing agricultural and energy output.
Panel density
For overhead agrivoltaic systems, the German Standards Organization (DIN) recommends that loss of agricultural land should not exceed 10% of the total area (13). Ideal configuration depends on crop type, but increased shading can significantly reduce the productivity of certain crop types and should be carefully considered to prevent adverse effects on crop health. Full density overhead systems (covering >75% of the plot) can be used for shade tolerant crops in all seasons. For medium or high shade-sensitive crops, panel density should range between 50-75% to ensure appropriate trade-off between energy and crop output (14).
For ground-mounted interspace systems, DIN recommends that loss of agricultural land should not exceed 15% of the total land area, to ensure the benefits of electricity production do not come at the expense of agricultural production (13). Interspace systems are typically less land efficient than overhead systems, as the area immediately under the panels is constantly shaded, which inhibits most crop types.
Row spacing
The appropriate amount of space between rows of solar panels varies greatly, depending on the crop type, among other factors. According to one study of overhead agrivoltaics, optimal row spacing for single-year long-term crops ranged between 7-11 meters (15). Leaving less space between rows would reduce crop productivity but increase solar electricity output.
Some crops can cope with panels being closer together. For some permanent perennial varieties (strawberries and other soft fruits), value per acre was significantly increased in agrivoltaic systems compared to open fields, even in high-density arrays (i.e., where solar panels cover >75% of the land) (16). However, rows should still be sufficiently spaced to allow harvesting machinery to access the crops.
In patterned or scattered arrays, panels are arranged to leave gaps in a consistent pattern. In a study observing lettuce in Spain, patterned arrays which created ‘scattered shade’ resulted in a 61.2% increase in fresh weight production during summer compared to ‘concentrated shade’ and a 87.6% increase compared to full sun exposure (17). Research into impacts of shade on agrivoltaic systems is ongoing and heavily dependent on crop type.
Crop placement
Different crops need varying levels of sunlight and humidity to grow, and planting each crop type strategically around solar panels is key to maximizing crop productivity and land efficiency. The figure below depicts three zones of land use surrounding ground-mounted panels. Zone 1 has the lowest level of light penetration (irradiance) and the highest level of humidity. This zone is therefore the least suitable for crops that require high sun exposure, for example (18).
Figure: Representation of Zones of irradiance and humidity in ground-mounted systems

Source: Toledo et al. (2021)
However, in one study of a particular pasture type (alfalfa), biomass increased by an average of 10% over two years due to shading from ground-mounted PV (19). Numerous studies have found that crops sensitive to reduced soil moisture benefit from the increased humidity surrounding ground-mounted panels (19).
Usage
Agrivoltaics is a practice that is already used extensively across the world, particularly in the UK and Uruguay for grazing sheep under solar panels (3). Government subsidy programs are increasingly being used to enable industry growth in Japan, China, France, and the US, which quadruped global megawatt capacity between 2012 and 2021 (Fraunhofer 2). In the US, the American Solar Grazing Associations rent sheep to solar companies for vegetation maintenance (3). By 2027, global agrivoltaic capacity is expected to reach 36,317.4 megawatts, a 45% increase from today (24).
Impact
Climate impact
Targeted emissions sources
Mixed land use facilitates emissions reductions across:
Scope 1 (for farmers who transition to mixed land use): Agrivoltaics can help reduce GHG emissions associated with fuel consumption. Electricity generated on site can also be used to power company vehicles.
Scope 2 (for farmers who switch to mixed land use): Agrivoltaics facilitate on-site production of renewable energy, which can reduce consumption of fossil fuel-based electricity in agricultural production and associated emissions.
Scope 3 (for farmers who transition to mixed land use):
Category 1: Purchased goods and services: Ammonia, a key ingredient in feed and fertilizer, is typically made using fossil fuels.If a farm uses on-site renewable energy to produce ammonia/feed/fertilizer instead of procuring fossil fuel-derived products, there would be a reduction in emissions relating to purchased goods (9).
Category 4: Upstream transportation and distribution: If a farm uses agrivoltaics to produce its own fertilizer on-farm, rather than transporting it from external suppliers, there would be a reduction in transport emissions.
Scope 3 (for food companies):
Category 1: Purchased Goods and Services: Agrivoltaics reduce land use change and facilitate increased use of renewables during crop production, reducing value chain emissions for customers purchasing these crops.
Decarbonization impact
Expected Outcomes:
Although only a few lifecycle assessments (LCA) have been conducted to determine if agrivoltaics are environmentally beneficial, studies have shown that they can yield reductions of up to 69.3% in GHG emissions from direct actions (Scope 1) and 82.9% in demand for purchased fossil fuel generated energy (Scope 2) when compared to separated food and solar farm production (39).
As 22% of agricultural emissions stem from energy use (1), agrivoltaics will help drive decarbonization throughout the agricultural sector, by providing renewable electricity that can be used on the farm.
Solar panels work better in colder conditions, and growing crops around panels reduces their temperature. As such, solar panels within agrivoltaic systems are often more efficient than those in standard solar parks (26).
Business impact
Benefits
Increased agricultural productivity: Farms using agrivoltaics for shade-tolerant crops have reported a 30% increase in economic value compared to farms deploying conventional agriculture (27). Many studies have also reported increased yields in crops produced using agrivoltaics (9). Shade provided by solar panels influences air temperature, direct sunlight, and water demand, which generates increased productivity compared to traditional open sky planting systems (26)(28). Studies suggest that productivity could increase globally by 35-73% for some crop types (9).
Additional revenue streams for farmers: Agrivoltaics diversify the use of agricultural land to create extra income streams. Renewables can also be built on unusable or less productive land. Farmers can also sell renewable energy back to the grid, or lease production rights to third parties. This provides a dual income to farmers under economic stress due to the unpredictable climate. In the US alone, less than half of farmers made a profit in 2018, which reflects a general need to supplement income (29).
Improved brand image: Products made using agrivoltaicsappeal to customers who are increasingly conscious of the environmental impacts or footprints of products.
Increased resilience to the impacts of climate change: Agrivoltaics reduce reliance on external sources of energy for food production, which is a prevalent concern in the current geo-political climate. Increased productivity through shading can reduce the impact of rising temperatures and changes in seasonal extremes, which have a direct impact on the prosperity of the agricultural sector and food security.
Costs
Investment required: Installing agrivoltaic systems incurs large upfront capital costs per acre, which may require external investment or government subsidization
Continued investment into R&D is needed to reduce the upfront cost of agrivoltaics, which is currently considerably higher than standard ground-mounted solar panels (30)
Average payback period is around 4-8 years
Relevant dependencies: In some countries, regulations place restrictions on the construction of projects in rural green belts, which could limit uptake (31)(32)
Impact beyond climate and business
Co-benefits
Animal Welfare: Solar panels and other renewable energy infrastructure provide protected areas of shade and windbreaks for grazing animals, leading to:
Potential side-effects
Research on crop productivity is mostly focused on warmer climates. The influence of increased shade on agricultural output is likely to vary dependent on region and could reduce productivity of crops that are not conducive to shaded areas (37)
Crop productivity in agrivoltaic systems depends on the layout of the system. In some cases, growth under the panels reduced by 84% but rose by 38% between panels when compared to conventional systems (37)
Creating agrivoltaic structures over larger livestock may increase costs and emissions intensity, and therefore reduce the perceived benefits to climate mitigation and economic output
Implementation
Typical business profile
Agrivoltaics are most suitable for farmers or companies with access to agricultural land. However, companies with access to flat commercial or industrial rooftops can also implement rooftop agrivoltaic systems.
Approach
Check for agrivoltaic initiatives in your region. They may be able to provide end-to-end technical assistance or advise on available funding. For example, in Germany, Fraunhofer provides support with designing, engineering, operating, and maintaining agrivoltaic systems that comply with the German Renewable Energy Sources Act and its associated financial incentives (8).
Choose whether to purchase your own solar panels or lease your land to a solar developer. If purchasing your own panels, you should look for grants to cover upfront costs and arrange an engineer visit to advise on plans. You’ll need permits to install the panels and connect them to the grid. If working with a developer, you should understand the value of your land and how close it is to the electricity grid, as well as the specifics of the lease. A developer may also obtain necessary permits for you (23).
Understand your requirements. The most important factors to consider are:
Crop and/or livestock type: Each crop type has different growth patterns and requirements for shade, moisture, and space, which will influence the setup of an agrivoltaic system. Similarly, the size and type of livestock will inform how solar panels should be arranged and whether supporting structures need to be strengthened. Each of these factors can influence cost and the relative productivity of renewable energy compared to agricultural production. You should also consider how crop care and harvesting will be impacted by agrivoltaic systems, including whether large equipment needs to move between rows of solar panels.
Land: Start by identifying which areas of land are best suited to support agrivoltaic structures. Due to physical or legal factors, some sites may not be viable.
Budget: More complicated structures and arrangements of solar panels will be more costly. Consider upfront costs relative to the rate of return from electricity generation (or additional revenue from increased agricultural productivity) to understand the feasibility of the project.
Electrical connection: Check if the farm is close to existing power lines that can be easily connected to the panels to use the electricity generated.
Design the agrivoltaic system to meet your requirements (see implementation and operation tips for more information). You or your consultant will need to determine:
The type and number of solar panels you need
Whether to have an overhead or ground-level system
How to arrange the solar panels
Stakeholders involved
Agrivoltaics associations: For example, Solar Power Europe provides best practice guidelines for agrivoltaics through their initiative, Agrisolar Europe. In France, major solar developers partnered in 2021 to create the world’s first trade body for the agrivoltaics industry. In the US, Agrisolar Clearinghouse connects farmers and developers and provides technical assistance and information on funding.
Solar developers: Farmers can choose to lease land to developers, who will install solar panels and pay farmers for the electricity generated.
Consultants/engineers: Engineers and consultants can provide end-to-end support for scoping, designing, and operating a system. They can provide advice for optimizing the system, including which type of panels to use.
Installers: You can choose to install your own solar panels or work with a qualified installer. If you are working with a solar developer, they will likely also carry out installation.
Insurers/financiers: As well as government grants, loans, and feed-in tariffs, you can purchase renewable energy insurance for your agrivoltaic system.
Lawyers: Legal professionals can help navigate land use restrictions and identify other legal barriers.
Local building authorities: Local authorities grant permission to build and connect agrivoltaic systems to the grid. They may need to inspect the specific layout of the system (23).
Local grid network providers: Network providers can advise on how to use or sell any excess energy you produce. It is important to consider these options early in design to increase the profitability of energy generation. There are several options, including:
Net metering, where excess energy is stored in the grid in return for credits. These credits can be used to offset periods of lower solar output.
Installing batteries alongside the panels, enabling you to store energy for later use. This will incur additional upfront investment costs.
Selling excess energy directly to the grid or allowing community members to purchase electricity (usually at a lower cost to conventional grid supply).
There may be additional costs involved in consulting some stakeholders, but their support to optimize agrivoltaic systems should lead to a return on investment.
Key parameters to consider
Solution maturity: Readily available, although not widely integrated. Market investment is growing and agrivoltaic infrastructure is expected to become a $9.3B market by 2031 (38).
Lifetime: Around 25-30 years
Technical constraints or pre-requisites: Some farming practices, like cut-and-burn farming, are not compatible with agrivoltaics. Planning permission is often required to install agrivoltaic systems. Systems can also take several months to install, and some farms may not be able to stay operational during this time.
Eventual subsidies available: Grants, loans, feed-in tariffs, and other incentives are available in different regions, including the US, China, France, Japan, and South Korea (8).
Implementation and operations tips
Designing an agrivoltaic structure to meet your requirements involves:
Calculating the array size
Start by calculating the amount of electricity you want to generate (in kilowatt hours). This is known as the system size. This could be enough to power all farm activities, or to sell excess energy to the grid. Then use this figure to calculate the number of panels required.
Use the following equation:
Number of panels = system size/production Ratio/panel Wattage (12)
System size = the total electricity production required (kilowatt hours)
Production ratio = how efficient the solar panel is, determined by the estimated energy output (kwh) over time for a specific system size. Production ratio varies depending on regional differences in climate which can alter the productivity of a panel. A higher ratio means that fewer panels are required to achieve the same electricity output compared to a lower ratio zone. Typical ratios fall between 1.1 – 1.7 (12).
Panel Wattage (or power rating) = the electrical output of the solar panel under ideal conditions. Typically, panel wattage falls within 300 – 400 watts (12).
NB: the production ratio of a solar panel in an agrivoltaic system is likely to be higher than in other conditions, due to the beneficial cooling effects provided by the companion crops.
Choosing the type and configuration of solar panels
The crop, livestock, land, and budget requirements you identified will inform the design of the agrivoltaic system.
Ground-mounted interspace vs. overhead structures: Some farm systems will have the capacity to support both; however, this will increase costs.
Panel type: There are a range of solar panel technologies to choose from. Semitransparent panels help reduce shade, which can benefit crops, albeit at the expense of some energy output. Bifacial systems can increase efficiency by absorbing light from both sides of the panel (including light reflected from the ground). These systems can improve efficiency of energy output by up to 24% (20).
Appropriate structure height: This will be determined by the height of the crops and/or livestock that will live alongside the panels. Refer to technical guidelines such as the DIN-SPEC 91434, which outlines criteria for optimal design height (21).
Appropriate panel tilt: This will be determined by the activity on the site, as well as wind direction, typical weather conditions, direction of peak sunlight, and panel module size (21). As well as traditional panels angled at 30⁰, consider using vertical systems or dynamic array structures that can move and tilt at different angles. Studies suggest that technologies that tilt the direction of a solar panel along one axis (single axis trackers) can increase the electricity yield of agrivoltaic systems by 22-26% (22).
Optimal row distance: Rows should be adequately spaced to ensure machinery and farm workers can continue to operate unhindered (21). Exact row distance will be determined by the optimum ratio of energy and agricultural output.
Configuration of panels: Although solar panels create additional shade, impacts on agricultural output can be minimized by using patterned arrays or reducing the density of arrays. Shade-tolerant crops should be placed in zones with less light exposure and vice versa.
Suitable plots of land: Ensure the system does not interfere with natural water supplies or disturb soil composition or compaction, as this can negatively impact crop productivity (21).
Electrical risks: Farm operations and livestock can damage solar infrastructure, meaning there is a higher risk of fire or electric shock in agrivoltaic systems compared to other solar arrays. Adopt electrical safety principles and pay attention to risks when designing and managing agrivoltaic systems (21).
Appendix
The table below shows the approximate number of solar panels required to achieve a certain system size and the expected output of a region with a relatively high production ratio (1.5).
System Size (kW) | Number of Panels Needed | Estimated Annual Production (kWh) |
4 | 10 | 6,000 |
6 | 15 | 9,000 |
8 | 20 | 12,000 |
10 | 25 | 15,000 |
12 | 30 | 18,000 |
14 | 35 | 21,000 |
The table below shows the typical spatial requirements (in square feet) for different system sizes and panel wattages:
System Size (kW) | Spatial requirements for 300 W panels (Sq. Ft) | Spatial requirements for 340 W panels (Sq. Ft) | Spatial requirements for 360 W panels (Sq. Ft) | Spatial requirements for 400 W panels (Sq. Ft) |
4 | 234 | 207 | 195 | 176 |
6 | 351 | 310 | 293 | 264 |
8 | 468 | 413 | 390 | 351 |
10 | 585 | 517 | 489 | 439 |
12 | 702 | 620 | 585 | 527 |
14 | 819 | 723 | 683 | 615 |