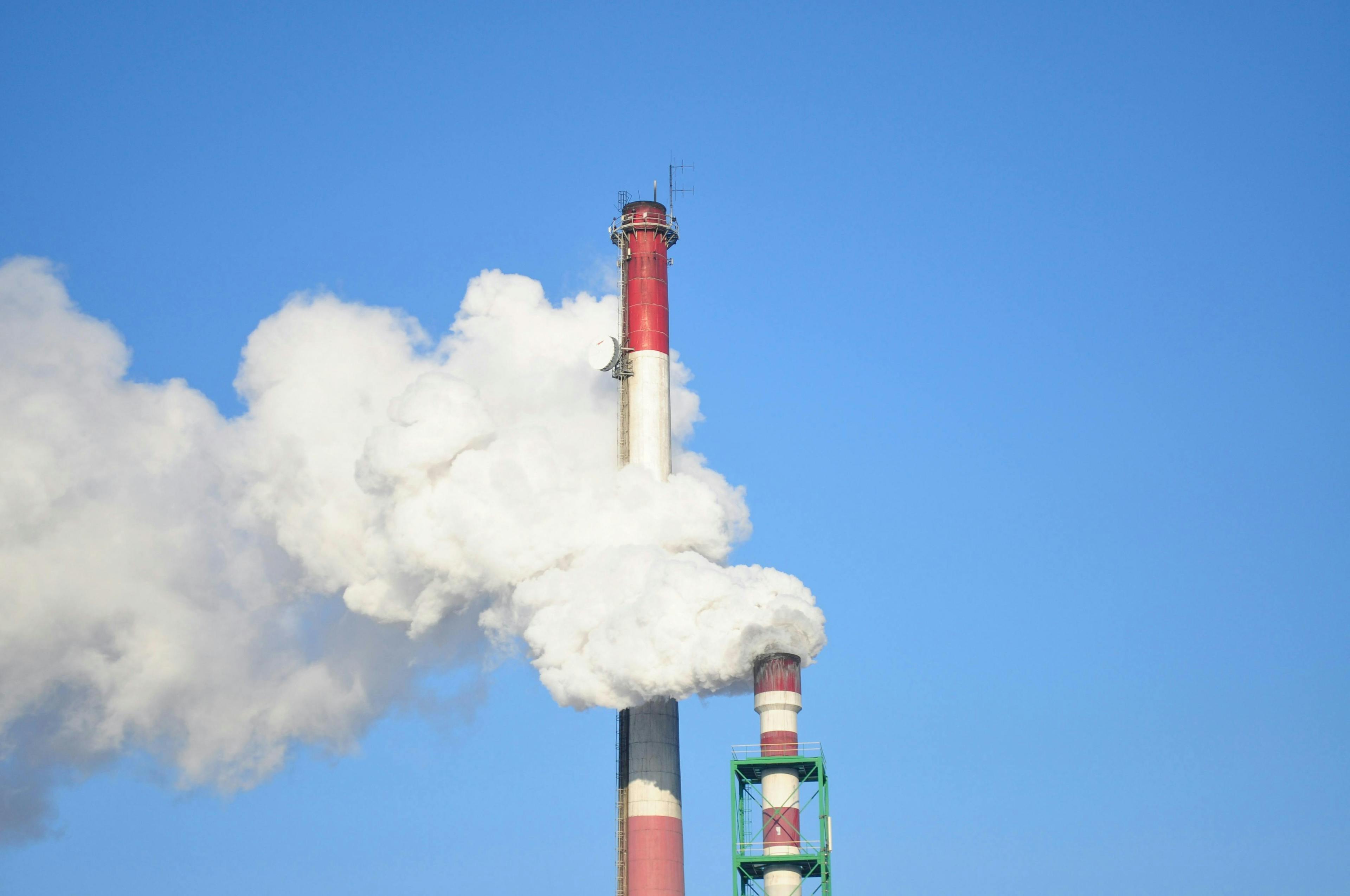
Adopt BECCS technologies
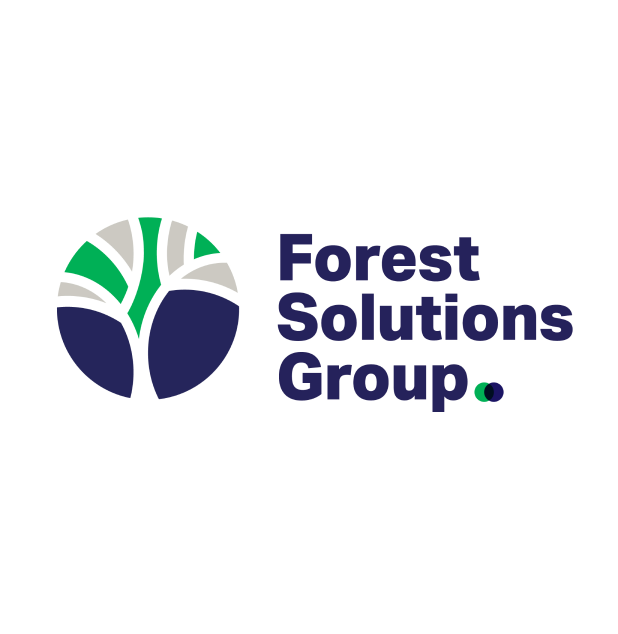
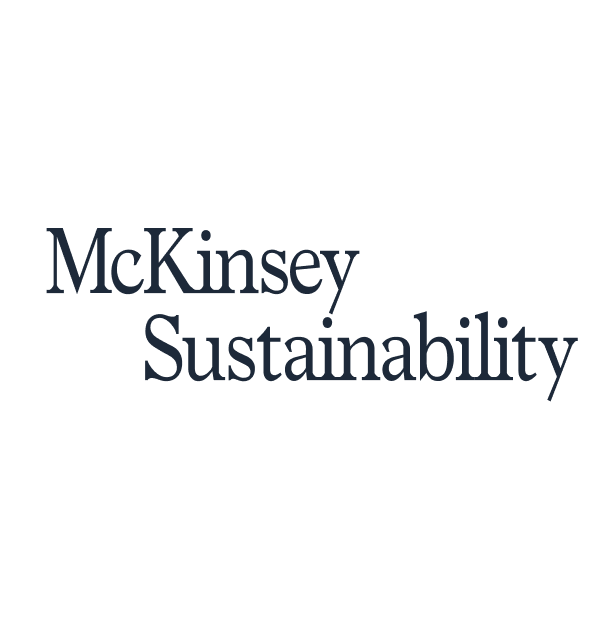
Summary
As the pulp and paper industry is the largest industrial consumer of biomass, there are many large sources of biogenic GHG emissions that could be captured with BECSS
Key resources
Context
This Action is one of ten from the Catalogue of Key Decarbonization Actions, part of the Forest Sector Net-Zero Roadmap. Its objective is to support forest companies in the choice and implementation of actions to address emission hotspots and leverage the greatest opportunities for carbon removals.
LEVEL OF MATURITY | EMISSION ABATEMENT POTENTIAL | SHORT-TERM ECONOMIC FEASIBILITY | |
---|---|---|---|
MEDIUM TO HIGH | HIGH | MEDIUM TO HIGH | |
• Large prototypes have been validated, larger-scale deployment is yet to be achieved • Some carbon capture technologies have achieved maturity • TRL 5 – 9 | • Nearly 100% of biogenic emissions from combustion can be removed | • Abatement cost: 125 – 250 EUR/tCO2 • Excluding potential revenues from carbon credits or others |
See methodology in the appendix section
Solution
Most IPCC scenarios rely on carbon removal technologies to achieve the Paris Agreement's objectives. Bioenergy with carbon capture and storage (BECCS) is typically considered to be a particularly significant option. As the pulp and paper industry is the largest industrial consumer of biomass, there are many large point sources of biogenic GHG emissions that could be captured.
Bioenergy with carbon capture and storage (BECCS) involves any technology through which biogenic CO2 emissions produced (from biomass combustion, as well as from gasification, fermentation or any other process causing biogenic emission release) are captured and permanently stored, for instance in a geologic formation (1).
Figure 1: How BECCS works

Source: McKinsey
There are several technologies to capture biogenic carbon, which can be divided into:
Pre-combustion capture, when CO2 is removed from a gas mixture before combustion. This technology can only be applied in the mills using black liquor or biomass gasification or with lime kilns using biomass gasification.
Post-combustion capture, when CO2 is separated from exhaust gases created by burning fuels. Many technologies exist, but the most used one is capture through chemical absorption, using solvents such as amines, monoethanolamide (MEA) or aqueous ammonia.
Oxy-fuel combustion, a process that reduces fuel consumption by burning fuel using pure oxygen (or a mixture of oxygen and flue gas) instead of air, can also be used for carbon capture by removing the water vapour from the flue gas rich in CO2 it produces and storing this gas after purification and dehydration (2)(3).
BECCS can be implemented to capture biogenic emissions from the manufacturing process in the pulp and paper mill, as well as to capture emissions released into the atmosphere at forest products’ end of life. In the pulp mill specifically, BECCS can be implemented at multiple points, given that there typically are multiple CO2 sources. In a mill producing kraft pulp, these are often found in the recovery boiler, the lime kiln, and the biomass boiler (4) However, it will likely not be economical to apply carbon capture to all potential sources (5).
Following the capture process, the biogenic carbon-dioxide is stored. Plants can store the biogenic CO2 directly underneath the plant, otherwise the biogenic CO2 has to be transported. In this case, it is purified and pressurized to transform it into the liquid form, as to make the transportation more efficient, since liquified CO2 is much denser and requires less volume than gaseous form. After liquefaction, the carbon dioxide is stored in buffer tanks, and is then shipped to the final storage site through multiple modes – barge, pipeline, ship, train or road (6). Captured CO2 is injected into naturally occurring porous rock formations, for example, unused natural gas reservoirs, coal beds that cannot be mined, saline aquifers, or deep ocean injection.
Usage
Although several carbon capture technologies exist and integrating carbon capture into the production of pulp and/or paper was found to be technically feasible, technologies are mostly in the development stage with limited utilization in relevant environments (TRL 5), while others (such as amines for post-combustion capture) are much more mature and utilized (TRL 9). Globally, as of 2022, there are around 20 BECCS plants currently operational or planned, varying by type, capacity, and costs, as well as industries where the technology is used: cement, waste and energy and power, and some scattered applications in the pulp and paper industry in Sweden and Canada.
Impact
Climate impacts
Targeted emissions sources
Capture of biogenic carbon with BECCS has the potential to permanently remove or remove for longer periods, CO2 from the carbon cycle.
Emission abatement potential
The pulp and paper industry holds high potential to leverage carbon capture technologies to significantly mitigate emissions, as up to 75% of emissions from the pulp and paper industry arise from biomass combustion onsite, particularly for chemical pulping plants. These biogenic emissions are considered carbon neutral and can largely be removed through BECCS (7). Studies estimate that BECCS wider deployment in Europe could help sequester up to 60 MtCO2, of which 20 MtCO2 is just in Sweden (8).
The carbon efficiency of CCS technologies (i.e., the net carbon it captures) vary depending on the type of technology adopted and the type of flue gas, although most current technologies can achieve up to 90-95% efficiency in terms of carbon sequestration.
However, the overall impact on the pulp mill emissions will vary depending on the energy sources and configuration. Studies in Swedish pulp and paper mills, with bioenergy demand ranging between 3-5.3 GWh/year, found that the overall potential for emission reductions from BECCS application would range between 53-66% (9).
Additionally, trade-offs must be made between carbon sequestration efficiency, energy consumption and costs (10).
Business impacts
Benefits
Revenue opportunity:
Companies adopting BECCS can increase revenues by obtaining carbon credits for the sequestered carbon. Currently, carbon credits can be exchanged in voluntary carbon markets. However, compliance carbon markets may soon be able to accept credits from technology-based carbon removals (11).
Should carbon not be stored, as in the case of the carbon being sold to synthetic fuels producers, players implementing BECCS could still benefit from revenues derived from the sale.
Other profitability considerations:
The distance to a storage location or CCS hub is a crucial consideration for maximizing the economic profitability of BECCS. The greater the distance to storage site, the higher the costs for the transportation of the liquified carbon-dioxide. Some pulp mills may have a relative advantage over others due to their location. For instance, in Europe, mills with proximity to the North sea may benefit from accessible storage potential, whereas in the southern US, projects are more likely to consider land storage.
Pulp mills that are less conveniently located can still benefit from carbon capture by selling the liquified biogenic carbon-dioxide to producers of synthetic fuels, such as methanol. Although this option may not provide as much value as carbon sequestration and selling carbon credits on the market, it may still be a favourable option, as the monetization of carbon removals is uncertain and occurs in relatively illiquid new markets.
Costs
Investment required
As most BECCS projects are either under development or at the prototype stage, capital and operating costs may be subject to strong variations over time. Capital costs of BECCS technologies currently range between 19-22 EUR/tCO2 depending on the type of technology and capacity (i). Initial capital costs vary depending on whether the plant is already in place (as in the case of pulp mills) or if it is constructed from scratch (as for energy production purposes). For instance, the investment to incorporate BECCS in the Saint-Félicien Pulp Mill in Canada was around 8 million EUR (with a target to remove 30tCO2/day), while other projects in the energy sector cost up to 450 million EUR (12).
Operating costs
Operating costs (excluding transport and storage) are estimated to range between 30-90 EUR/tCO2, as they vary based on energy prices, which is the main driver of BECCS costs (13).
Indicative abatement cost
Studies suggest effective cost of carbon removal for BECCS range between 125-250 EUR/tCO2which are expected to decrease to 50-160 EUR/tCO2 when deployed at scale by 2050 (14). Other sources indicate lower costs, specifically for the paper and pulp mill, ranging between 40–90 EUR/tCO2 (15). Furthermore, the estimated abatement costs currently do not factor in the potential profits that could be generated from selling the sequestered carbon as either carbon credits or to markets and producers. If such additional revenues were taken into account, BECCS would appear a cost competitive option for the forest sector.
Potential co-benefits and side effects
Co-benefits
Energy generation coupled with carbon removal: BECCS technologies can also be used to couple energy generation from biofuels and carbon removal technologies to ensure both the supply of clean energy and climate change mitigation benefits.
Side effects
Trade-off between biofuels and land use
While most IPCC studies acknowledge the great potential of BECCS, they also warn of potential adverse environmental socio-economic impacts, as significant usage of BECCS would require a large amount of biomass. These potential drawbacks include changes to land use patterns that may increase GHG emissions, pressure on water resources, soil health, air and water pollution, and pressure on food availability and costs (16). As a bioenergy, BECCS is only considered sustainable when the sourced biomass is grown sustainably or based on waste or residues (17). Competition with other forms of land use and other external impacts should be taken into consideration and avoided when relevant. The EU Commission’s Renewable Energy Directive’s sustainability criteria for bioenergy encourages companies to source bioenergy from feedstocks with low indirect land use change risk and to minimize the use of whole trees and food and feed crops (18). However, these concerns can be mitigated when applying BECCS on top of the pulp and paper process, because BECCS is used as a side stream to producing materials, and not simply for the sake of storing carbon. This makes the pulp and paper industry well suited for BECCS.
Increased energy demand on site
The carbon capture process requires steam and electricity and may require up to an additional 25-30% of additional fuel and additional electricity consumption. Carbon capture will increase the demand for biomass or other energy sources, potentially resulting in further costs.
Carbon leaks and safety
There is a small risk that carbon sequestered into geological formations could leak out of the underground reservoirs and pollute air or taint nearby water supplies. However, geological storage has been proven to have low levels of leakage, if best practices are followed (19). Tremors may also occur due to the build-up of pressure underground, known as induced seismicity. However, such risks can be minimized by injecting CO2 into soft sedimentary rock formations, like shale or sandstone, as these are less prone to causing earthquakes (20).
Implementation
These are the most common steps to adopt BECCS technologies:
Select the appropriate equipment and process: The company must decide what type of technology to use and at which stage of the production process (or multiple, if applicable). This should be informed by existing processes (e.g. pre-combustion may be appropriate for a mill using black liquor), an assessment of technical feasibility and cost.
Adapt existing infrastructure and processes: Upgrade the existing operations by retrofitting BECCS infrastructure and adapt current production process, as appropriate, to use bioenergy and capture carbon, or establish new pilot mills fitted with BECCS technology.
Ensure adequate measurement and verification of captured carbon: The issue of carbon credits from BECCS may require a partnership with project developers and carbon credit verification organizations with expertise in the measurement of negative emissions, as well as in the monitoring, verification and issuance process.
Set up a CO2 value chain: Storing the captured carbon requires setting up a CO2 network or a connection with an existing CCS hub.
Key challenges/hurdles
Uncertain monetization of removals: The main carbon credit standards are still in the process of developing carbon accounting methodologies for the issue of carbon credits from technology-based removals like BECCS (21). Moreover, current climate change mitigation policy frameworks like the EU ETS currently do not provide incentives for negative emissions.
Uncertain policy: BECCS pilot and demonstration projects are generally still first-of-a-kind and therefore unproven, not because the technology is unknown but because the various components have not yet been combined repeatedly at scale. As a result, industrial policy is complex and still evolving, exposing companies to regulatory uncertainty.
First mover challenge: There are few incentives for industrial actors to be first movers and bear the high risk and infrastructure costs associated with setting up a CO2 transport network.
Potential solutions
Partner with carbon credit certification schemes: This may allow organizations to better understand emerging guidelines for the monitoring of captured emissions and issuance of BECCS carbon credits. Furthermore, partnerships may offer an opportunity to support the establishment of guidelines, ensuring the potential to monetize the application of BECCS in the industry.
Develop industry partnerships: Organizations could partner with technology providers and governments to act as pilot projects, which may provide access to equipment at a reduced rate and an opportunity to shape technology development to ensure it is fit for industry purpose. Additionally, partnerships with industrial clusters can help establish a lower cost transport and storage network.
Footnotes
(i) Note: McKinsey analysis
Going further
Check out other Actions as part of the Forest Sector Net-Zero Roadmap:
Appendix
Methodology for assessment against criteria
Level of maturity: The level of maturity score for each action is based on the ‘Technology Readiness Level’ (TRL). This indicator estimates the maturity of technologies, measured through an assessment of their progress and capabilities. The scale originally ranges from 1 to 9, where TRL 1 is the lowest and TRL 9 is the highest. When a technology is at TRL 1, scientific research is underway and results are being translated into future research and development, while at TRL 9 the technology has already been proven to work. The International Energy Agency (IEA) has extended the TRL scale used in this report to incorporate two additional levels of readiness: one where the technology is commercial and competitive but needs further innovation efforts for the technology to be integrated into energy systems and value chains when deployed at scale (TRL 10). Finally, there is a level where the technology has achieved predictable growth (TRL 11).
Emission abatement potential: The emission abatement potential of each action, whether a technology or practice, describes the potential to reduce GHG emissions with respect to the counterfactual technology or practice, meaning the technology or practice that is part of the ‘business as usual’ scenario, or that is substituted or improved by adopting a decarbonization action. Emission abatement potential is usually expressed as a percentage, and the higher it is, the higher GHG emission reductions can be achieved:
Low = < 15% of GHG emissions Medium = 15-50% of GHG emissions High = > 50% of GHG emissions
Abatement cost: The abatement cost is an indicator that measures the costs associated with abating one ton of GHG emissions (EUR/ton CO2e abated). The lower the abatement cost, the cheaper it is to reduce emissions, and therefore the more attractive the action.
Short-term economic feasibility: Economic feasibility is assessed based on the maturity level and the abatement cost of each action, as per the visualization below. Actions with a low maturity level (≤ 4) are considered to have low economic feasibility in the short term, given the need to validate and deploy the technology in relevant environments. However, if the abatement cost has already proven to be low, the action is assessed as medium. Actions with medium maturity (TRL 5-8) may have low or medium economic feasibility in the short term, depending on the abatement cost (≤ 250 EUR/tCO2e). Similarly, the short-term economic feasibility of actions with higher maturity (TRL 9-11) varies depending on the abatement cost (low when < 550 EUR/tCO2e, medium if the cost ranges between 250-450 EUR/tCO2e, or high if the cost is lower than 250 EUR/tCO2e). The thresholds that determine short-term economic feasibility are defined by taking into consideration that additional benefit, including revenue generating opportunities. They are not monetized or included in the abatement cost estimates.