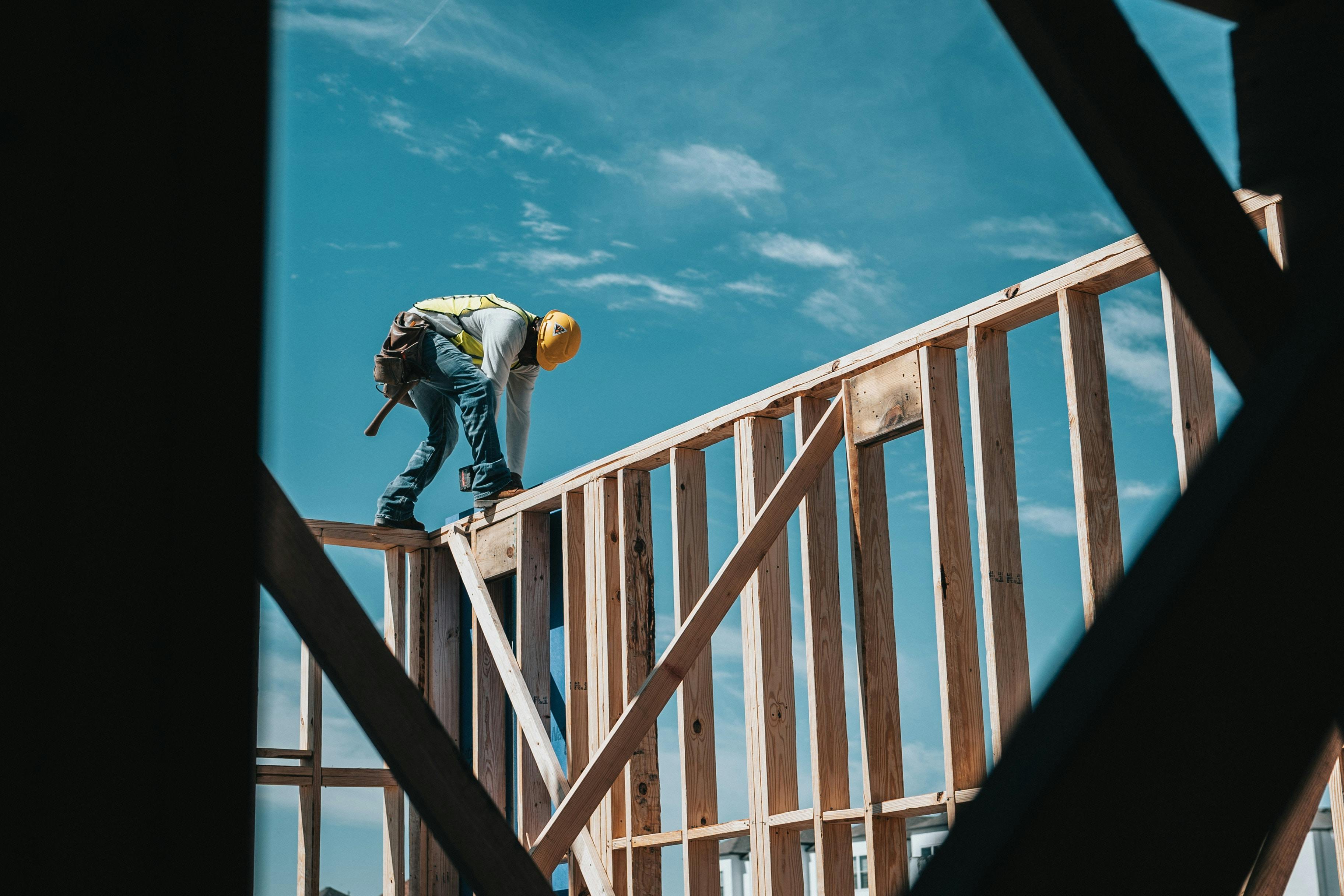
Adopt wood products to reduce construction emissions
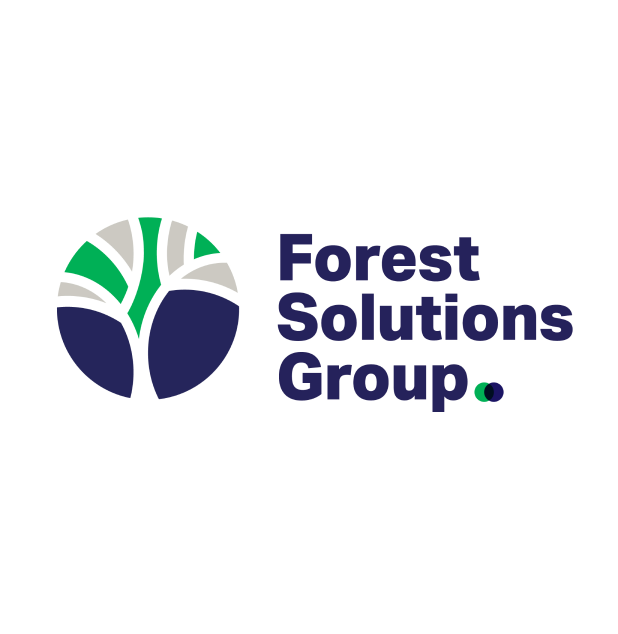
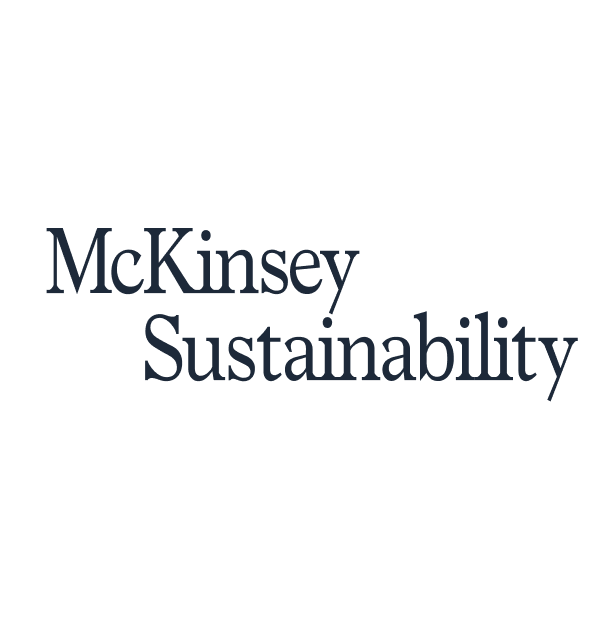
Summary
Using sustainably sourced wood products in construction can mitigate GHG emissions from the built environment while providing other benefits such as ease of installation.
Key resources
Context
This Action is one of ten from the Catalogue of Key Decarbonization Actions, part of the Forest Sector Net-Zero Roadmap. Its objective is to support forest companies in the choice and implementation of actions to address emission hotspots and leverage the greatest opportunities for carbon removals.
LEVEL OF MATURITY | EMISSION ABATEMENT POTENTIAL | SHORT-TERM ECONOMIC FEASIBILITY | |
HIGH | MEDIUM | HIGH | |
• High maturity, stability achieved • TRL 11 | • 20 – 30% emission reduction for a building | • CAPEX: 470 – 860 EUR/m2 • Abatement cost: 50 – 90 EUR/tCO2 |
See methodology in the appendix section
Solution
The forest sector can help mitigate GHG emissions from the built environment by promoting the use of solid wood and engineered wood products in construction. The built environment is responsible for approximately 40% of global energy-related carbon emissions (1), and a third of these are associated with materials and the construction process, or embodied carbon, primarily linked to concrete, steel, aluminium and plastic.
Scaling up the use of sustainably sourced wood products, such as solid wood and engineered wood products (see Box 1), can result in significant emission reductions in the built environment under the right conditions, and as part a broader set of decarbonization actions (2). Manufacturing of wood products is less energy-intensive than traditional concrete and steel buildings. Also, the overall carbon footprint of buildings can be further improved through reused and recycling at the end of life (3)(4). In addition to the climate benefits, solid and engineered wood products offer a wide range of benefits, including ease of installation, high strength-to-weight ratio, aesthetic features and more (see Potential co-benefits section). It should be noted that the climate benefits of substituting traditional materials such as concrete and steel with wood products can vary and should be assessed on a case by case basis.
Usage
Solid wood has a long history of use in the construction sector, as well as more innovative engineered wood products that are also linked to very high maturity levels (TRL 11).The market for wood-based construction materials is growing, as well as the global production capacity of cross-laminated timber that increased at a rate of 10% between 2019-2020 dueto the addition of large scale production facilities. This growth is projected to continue at a steady rate over the next years (i).
Despite the growth prospects, the adoption of wood products in the construction sector is hindered by low awareness levels related to their benefits over more traditional building materials. The perception that wood products have limited structural performance, fire resistance and durability is also still widespread, despite studies demonstrating the opposite (5)(6). The forest sector currently faces a unique window of opportunity to champion wood as a key action for decarbonizing the construction sector, as steel, cement, and other structural materials continue their decarbonization. Increased evidence of buildings using wooden structures, and more architects trained to adopt these products will contribute to overcoming these barriers to adoption.
Box 1: Engineered wood products: Trends in different product categories
Engineered wood products are wood products that are made by binding together fibres or boards of wood with adhesives. They are designed to respond to a particular specification, for instance, flooring or pillars.
Category | Product | Description (7) | Key trends (ii) |
---|---|---|---|
Structure and beams | Cross-laminated timber (CLT) | Multiple layers of solid saw lumber are glued perpendicularly for strength. It is very flexible in thickness and good for isolation. | CLT is a growing niche to replace concrete in floor and walls. |
Wood joists (I-joists) | I-shaped wood product that resists bending stress and carry heavy loads with less wood than a solid lumber joist. | This product is mature and already widely used for structural use in floor and roofs. | |
Laminated strand and veneer lumber (LVL) | A very strong material, but with strength in only one direction because all veneers have grains going in the same direction. | LVL has potential to replace concrete structures in framing. | |
Glulam | Multiple layers of dimensional lumber are glued together, with all grains running parallel to the longitudinal axis. It can also be produced in curved shapes. | This is a mature product, with the largest demand in Europe. It is used in load bearing structures such as bridges or canopies. | |
Wood panels | Particle and fibreboards, including Oriented Strand Board (OSB) | Wood strands and flakes are combined with adhesives and compressed in wide mats. | Large commoditized market for construction. OSB is mainly used for flooring, roof decking and wall sheathing in family housing. |
Medium/High Density Fibreboards (MDF & HDF) | Used in non-structural applications. Made from hardwood and softwood pieces broken down into fibers and pressed together at high temperatures to provide a smooth surface with high durability. | So far, MSF is used mostly for furniture and not construction, despite its appealing properties such as density and low cost. | |
Plywood | Thin cross-laminated veneer layers glued together, with grain direction is alternated from layer to later to maximize strength and stiffness. | Usage is growing in Asia, for instance for furniture, floors, ceilings, doors. |
Impact
Climate impact
Targeted emissions sources
The use of wood in construction, whether in the form of solid wood or engineered wood products, contribute to reducing GHG emissions associated with building materials and construction (embodied carbon). It also contributes to avoided emissions related to the substitution of higher carbon construction materials with wood.
Emission abatement potential
Carbon storage: In addition to GHG emissions reduction from embodied carbon, wood products also contribute to carbon storage. An estimated 50-70% of the carbon sequestered in the original tree is stored in wood products for the duration of their lifetime (8) representing approximately 700-800kg CO2 per cubic meter (9)(10)(11). However, it’s important to remember that when a wooden building is demolished, the stored carbon is released into the atmosphere, unless specific measures are taken, such as converting it into biochar or using CCS facilities. Addressing the end-of-life scenario is crucial also for players in the construction sector to be able to claim the carbon sequestration benefits, although different rules may apply depending on the local regulation or certification standard.
Avoided emissions: Around 20-30% of the construction emissions of a building could be avoided by substituting higher carbon building materials (e.g. cement, steel and aluminium) for wood, which is associated with lower embodied carbon emissions (12).
Box 2: Reducing emissions from glue in engineered wood products
Although solid wood and engineered wood products are generally more environmentally friendly compared to traditional construction material, the manufacturing is an emission hotspot in the value chain, and decarbonizing the manufacturing of engineered wood products is key to further improve their potential in the construction sector. A relevant proportion of GHG emissions in the manufacturing of engineered wood products is due to the use of glues and adhesives used to join together the components of engineered wood products. There is an opportunity to decarbonize through breakthrough technologies, such as biobased glues fabricated from lignin, which can substitute petroleum-derived glues. These types of products are currently under development and their large scale application will require further technical improvements (e.g. in water resistance and for bonding strength). However, they represent an appealing decarbonization opportunity to help progress the forest sector towards net-zero while unlocking further commercial opportunities and improving resource efficiency (13).
Business impact
Benefits
Reduced operating costs: The overall costs for constructing buildings with wood products can range from 20% less to 26% more than traditional options (14)(15). As wood is lighter than steel and concrete, less energy is required during the transportation phase and manipulation of wood at the construction site (although this varies across applications).
Lower operational costs are associated with the use of wood products (particularly due to lower maintenance costs), particularly when coupled with modular conception. This can reduce the overall cost of buildings by up to 20% (16). Additional savings can be made through reduced labour costs, as wood based units can be built up to 50% more rapidly, compared to traditional buildings (17) and up to 75% less workforce is required to build simple structures (18).
Costs
Investment costs in building materials: Depending on the type of product, prices can be slightly lower or higher compared to traditional construction materials, such as steel and concrete (which prices range from 410-750 EUR/m3) (19)(20). For instance, glulam beams can cost ~400-600 EUR/m3, while CLT prices range from 450-900 EUR/m3 (21)(22). Ultimately however, the costs of wood-based products for construction depend not only on the type of product, but also on the performance characteristics, the country and local market size, and the deals in place with manufacturers.
Indicative abatement cost: The abatement cost for the increased use of wood in construction, such as cross-laminated-timber, is estimated to be 45-90 EUR/tCO2 (23), though it may be significantly more. Cost varies depending on regional prices of wood, concrete and steel, and the quantity of wood used in a structure to replace a given quantity of steel or concrete.
Potential co-benefits and side effects
Co-benefits
Reduced water consumption in production: Mass timber production requires 30 times less water per cubic meter than reinforced concrete, which represents an additional sustainability benefit (24).
Improved energy efficiency in buildings: Buildings using CLT tend to have better energy efficiency in mild climates compared to ordinary buildings due to mass thermal effects balancing heat gains and losses (25).
Seismic resistance: Wood-engineered products were found to have a higher seismic resistance, a valuable characteristic in earthquake prone regions (26).
Side-effects
Material properties: While wood products are widely used in construction due to their sustainability, affordability, and aesthetic appeal, they are not without their downsides, especially in terms of structural properties. One significant drawback is their vulnerability to decay, pests, and fire when not properly treated or maintained. This vulnerability can be more pronounced in humid environments, potentially leading to structural integrity issues and necessitating costly repairs or replacements. Additionally, wood products typically have lower load-bearing capacity, limiting their suitability for high-rise buildings or in seismic regions (27). From a technical standpoint, wood-based products cannot entirely replace traditional construction materials like steel and concrete. For instance, most new buildings will require concrete foundations, and wood may not be suitable for certain architectural designs. This means that the emission reduction potential of wood products is inherently tied to these limitations and the advancements in product and architectural design.
Environmental and social risks: Where there is a lack of regulatory and voluntary control mechanisms, there is a risk of exacerbated environmental and social risks associated with timber production and harvesting, if implemented unsustainably. Increase in demand may lead to an increased risk of forest conversion and degradation, without appropriate safeguards. It can also present social and economic implications for local communities, as land tenure conflicts are common in some regions (28). However, many of these risks can be mitigated by using sustainable forest management practices, investing in assurance through forest certification programs, and improving forest management regulation and oversight from governmental bodies.
Implementation
The forest sector can support the transition to a low carbon economy by promoting the use of wood products in the construction sector. These are the most common steps that organizations can take to increase the adoption of wood products in construction:
Steps for construction companies
Assess structural requirements: Before incorporating wood products into construction projects, assess the structural requirements of the construction project. Structural engineers and architects will determine which wood products can meet structural considerations, such as necessary load-bearing capacities.
Identify and engage with suppliers that apply the highest standards of sustainable forest management: Companies should prioritize sourcing wood that is certified by globally recognized forest certification systems such as FSC, PEFC and SFI. These certifications verify that forest management practices meet stringent environmental, social, and economic criteria, ensuring the production of sustainable and responsibly sourced wood for construction.
Build long-term relationships with suppliers: Building relationships with suppliers who specialize in forest products will help construction companies access a wider range of materials and co-develop customized solutions.
Steps for forest companies
Harvest suitable trees, and invest in technology for improved processing: Forest companies can harvest trees that possess desirable characteristics for construction, such as straight grain, strength, and density. They can also invest in optimizing wood processing methods. This includes utilizing modern sawmilling techniques, drying technologies and manufacturing processes to produce consistent and high quality wood products suitable for construction.
Collaborate with downstream partners: Forest companies can collaborate with other stakeholders in the value chain, including architects, engineers, builders, and manufacturers. This collaboration fosters knowledge sharing, promotes innovative design and construction practices, and ensures the availability of wood products that meet industry requirements.
Key challenges/hurdles
Lack of incentives and regulation: Worldwide, there are few financial incentives for the construction sector to use wood products in construction. Furthermore, stringent low carbon building standards are only just emerging in leading countries.
Uncertified timber products: The absence of certification for some timber products may disincentivize their adoption in the construction sector in regions that present higher risks.
The absence of a broadly recognized and credible accounting framework for avoided emissions: This has so far disincentivized forest companies from quantifying or disclosing avoided emissions from their product portfolios to capture additional value from the climate-related benefits of wood products.
Potential solutions
Promote the benefits of using wood products: Raise awareness among architects, engineers, builders, and other stakeholders on the renewable nature, carbon sequestration potential, energy efficiency, versatility and design possibilities associated with the use of wood.
Support research and education: Invest in research initiatives and support educational programs focused on sustainable wood construction, such as research projects, scholarships and fellowships that advance knowledge in wood engineering, design and construction.
Engage with policymakers to promote incentives for the use of wood products: Engage with government agencies, policymakers, and industry associations to advocate for financial incentives, grants, or tax benefits to create favourable economic conditions for wood-based construction projects. Explore partnerships with financial institutions to develop specialized financing options for sustainable construction projects using forest products.
Engage with carbon accounting organizations to develop an accounting framework: Co-developing a framework can provide clarity around disclosure of avoided emissions associated with product portfolios, potentially stimulating demand among construction companies with decarbonization targets.
Footnotes
(i) Note: McKinsey analysis based on American City & County; CityLab; WoodWorks, Science Direct
Going further
Check out other Actions as part of the Forest Sector Net-Zero Roadmap:
Appendix
Methodology for assessment against criteria
Level of maturity: The level of maturity score for each action is based on the ‘Technology Readiness Level’ (TRL). This indicator estimates the maturity of technologies, measured through an assessment of their progress and capabilities. The scale originally ranges from 1 to 9, where TRL 1 is the lowest and TRL 9 is the highest. When a technology is at TRL 1, scientific research is underway and results are being translated into future research and development, while at TRL 9 the technology has already been proven to work. The International Energy Agency (IEA) has extended the TRL scale used in this report to incorporate two additional levels of readiness: one where the technology is commercial and competitive but needs further innovation efforts for the technology to be integrated into energy systems and value chains when deployed at scale (TRL 10). Finally, there is a level where the technology has achieved predictable growth (TRL 11).
Emission abatement potential: The emission abatement potential of each action, whether a technology or practice, describes the potential to reduce GHG emissions with respect to the counterfactual technology or practice, meaning the technology or practice that is part of the ‘business as usual’ scenario, or that is substituted or improved by adopting a decarbonization action. Emission abatement potential is usually expressed as a percentage, and the higher it is, the higher GHG emission reductions can be achieved:
Low = < 15% of GHG emissions Medium = 15-50% of GHG emissions High = > 50% of GHG emissions
Abatement cost: The abatement cost is an indicator that measures the costs associated with abating one ton of GHG emissions (EUR/ton CO2e abated). The lower the abatement cost, the cheaper it is to reduce emissions, and therefore the more attractive the action.
Short-term economic feasibility: Economic feasibility is assessed based on the maturity level and the abatement cost of each action, as per the visualization below. Actions with a low maturity level (≤ 4) are considered to have low economic feasibility in the short term, given the need to validate and deploy the technology in relevant environments. However, if the abatement cost has already proven to be low, the action is assessed as medium. Actions with medium maturity (TRL 5-8) may have low or medium economic feasibility in the short term, depending on the abatement cost (≤ 250 EUR/tCO2e). Similarly, the short-term economic feasibility of actions with higher maturity (TRL 9-11) varies depending on the abatement cost (low when < 550 EUR/tCO2e, medium if the cost ranges between 250-450 EUR/tCO2e, or high if the cost is lower than 250 EUR/tCO2e). The thresholds that determine short-term economic feasibility are defined by taking into consideration that additional benefit, including revenue generating opportunities. They are not monetized or included in the abatement cost estimates.