Optimize sorting & recycling for paper and textile
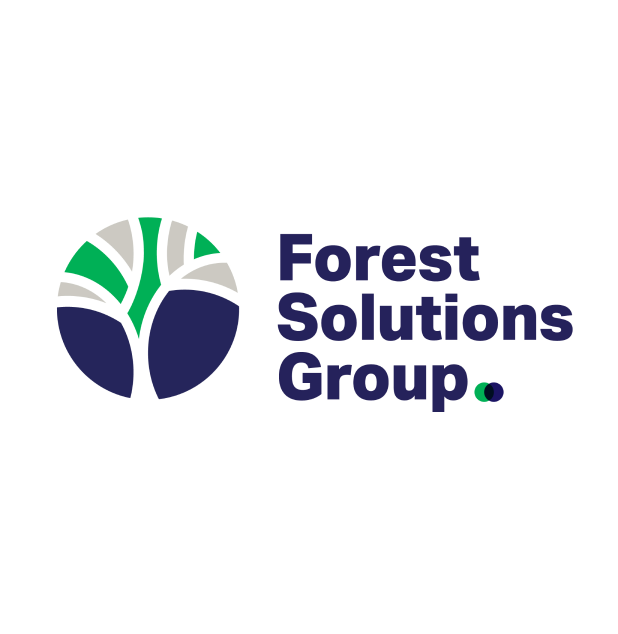
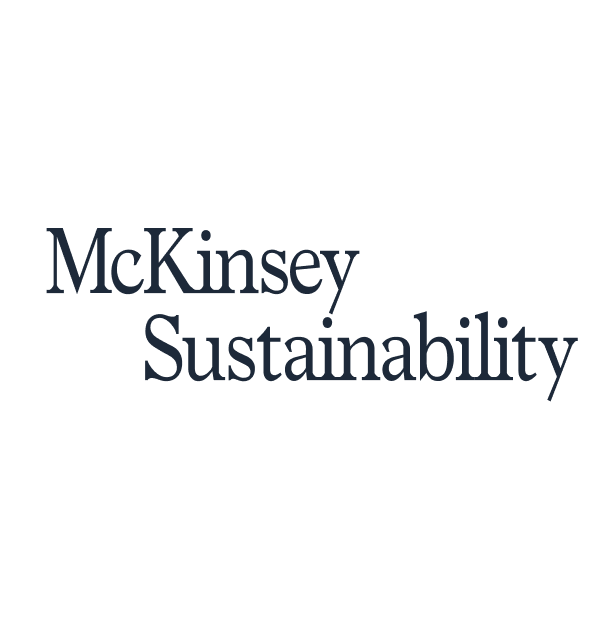
Summary
Adopt sorting technologies combined with improved design and better integration of recovered paper into manufacturing processes can reduce GHG emissions improving resource efficiency.
Key resources
Context
This Action is one of ten from the Catalogue of Key Decarbonization Actions, part of the Forest Sector Net-Zero Roadmap. Its objective is to support forest companies in the choice and implementation of actions to address emission hotspots and leverage the greatest opportunities for carbon removals.
LEVEL OF MATURITY | EMISSION ABATEMENT POTENTIAL | SHORT-TERM ECONOMIC FEASIBILITY | |
---|---|---|---|
MEDIUM TO HIGH | MEDIUM TO HIGH | MEDIUM HIGH | |
• First-of-a-kind commercial, adoption in relevant environments | • Depends on technologies, practices adopted and the material recycled (30 – 90%) | • Based on the type of technology and practice adopted |
See methodology in the appendix section
Solution
The recycling of paper products and cellulose-based textiles faces several challenges that hinder their reuse. For paper products, collection and recycling is a well-established practice, with a collection rate of 80% and a recycling rate of 60%. However, several production processes could lead to contamination, presenting significant challenges to achieving higher recycling rates. Examples of such processes where different paper grades need to be sorted and separated include disposing waste into single waste bins and collecting streams collectively (instead of separately). This can be challenging for the sector as sector, fabrics with multiple types of fibers are difficult to sort, and the process is reliant on manual labor, leading to low recycling rates. Additionally, recycling technologies often do not produce fibers of sufficient quality for textile applications. To increase the overall recycling rate of paper and textile products, potential solutions include improving product design to facilitate recycling, increasing the capacity to integrate recovered paper into manufacturing processes, and sorting technologies for mixed waste management. The latter solution holds particular promise for achieving higher recycling rates (see below).
Sorting technologies (for paper and textiles)
Sensor-based technologies have shown high potential for sorting paper and textile products. For instance, near-infrared technologies, such as hyperspectral imaging and visual spectroscopy use sensors and infra-red to detect material composition and colors. They can even employ artificial intelligence through algorithms and machine learning to continuously improve sorting performance. Through high yield and purity sorting, mixed paper can be effectively used. These optical sensors have proven to be particularly effective, with some infra-red methods achieving 100% accuracy in polymer material detection and classification (also by color), and may be able to scan up to one garment per second (1)(2).
Beyond this, through digital watermarking, imperceptible codes can be applied on packaging, which can be detected by high resolution cameras. Packages can then be sorted into different streams more efficiently, due to the information embedded in the code (e.g. material composition). Similar initiatives exist, such as including information in the bar codes for paper packaging or using a ‘digital passport’ for clothes (3). For textiles, adding disassembling microwave technologies to the sorting process could be also helpful: these technologies use microwave radiation to remove labels from clothes and fasten the preparation of textiles for the recycling process (4).
Textile recycling technologies
Textile recycling technologies fall into four primary technology categories (see Table 1). These differ in terms of energy efficiency and their ability to maintain or restore virgin quality of textiles. Typically, there is a trade-off between the ability to return to virgin quality and energy efficiency, which are counter-correlated. This creates challenges in producing high quality materials through recycled textile waste in an energy (and cost) efficient way.
Table 1: Technologies to improve textile recycling
Mechanical recycling | Mechanical recycling uses physical forces such as cutting and grinding to convert textiles into usable fibers. It is a commercially proven, low energy and cost-efficient recycling method. This technology currently faces the challenge of the quality degradation of recycled fibers with a fiber-length reduction of up to 30 to 40%, limiting its application and potential for upcycling. However, companies are exploring higher quality mechanical recycling and other innovative solutions. |
Soft mechanical recycling | Soft mechanical recycling is a process that maintains fiber length thanks to an innovative production line. The longer fiber length minimizes losses from the subsequent spinning process, overcoming the typical challenges of mechanical recycling. |
Thermo-chemical recycling | Thermo-chemical recycling uses gasification to produce syngas through the partial oxidation reaction of polymers, and is compatible with all forms of fibers. Thermo-chemical recycling as a core technology exists at a commercial scale, however, this technology needs some adaptation or development for the treatment of textile waste. |
Chemical recycling | Chemical recycling is a broad category of multiple distinct technologies that use chemical processes to break down fibers to the polymer or monomer level. Chemical recycling targets a broad set of fiber types including synthetic cellulosic fibers. |
Usage
Sensor-based sorting technologies have reached a decent level of maturity for improved sorting in the textile recycling industry, with some companies already selling machines to aid in this process (TRL 9).
Textile recycling technologies are used to varying degrees depending on the method and on the type of fiber. The majority of the technologies are still in the demonstration stage, requiring further development to increase the quality of the outputs while reducing costs (TRL 6-8).
Currently, the use of both sorting and recycling technologies is limited because of the high capital investment required and the lack of incentives in market pricing for recycled products. In fact, it is often more convenient and cost-effective for manufacturers to purchase virgin materials or to ship waste to other regions of the world. For instance, in the Netherlands, 55% of textile sorting takes place abroad (5).
Impact
Climate impact
Targeted emissions sources
By promoting the adoption of innovative technologies for improved sorting and recycling of paper products and textiles, the forests sector can reduce emissions from both the end of life – emissions due to products being discarded in landfills or combusted without energy recovery – as well as by increasing resource efficiency, therefore avoiding GHG emissions associated with the production of virgin fibers.
Emission abatement potential
Adopting sensor-based technologies and therefore increasing the recycling rate of paper products can reduce emissions of paper products by an estimated 30% (i) This is however dependent on the energy and resource efficiency of each mill, as those with high efficiency can produce lower emission products from virgin fibers compared to those using recycled fibers (6)
For textile recycling, mechanical recycling technologies, for example, offer a 60 to 90% GHG emissions reduction, as mechanically recycled fibers also avoid emission-intense, post-material processing, which saves more GHG emissions. Chemical pulping recycling of cellulose-based textiles has a lower potential to reduce GHG emissions compared to virgin fibers and the magnitude of the savings varies widely in different estimates and is still the subject of scientific evaluation (7). However, and despite the emission reduction potential, many textile recycling technologies cannot yet prevent a loss in fiber quality. The ’downcycling’ of fabric and fibers ultimately limits their market and therefore the potential for emission abatement.
Business impact
Benefits
Opportunity to increase revenues due to growing demand for recycled products: Consumers are increasingly demanding sustainable products. For instance, searches for sustainable fashion brands increased by 600% between 2016 and 2020. There is a business case for recycling paper packaging and textiles: if scaled, recycling of textiles could create a global $6-12 billion pool of value by 2030 (8).
More efficient processes in paper mills: Optical sensors can help improve energy efficiency in paper making. In fact, recovered paper sorting automate recycled feedstock sorting and deliver a more efficient deinking process that leads to electricity and steam savings of 16% and 30% respectively, and a reduction of 20% in material loss compared to a typical plant (9).
Costs
Investment required:
Sensor-based sorting technologies: Optical sensors machines optimized with artificial intelligence could cost between 200,000-570,000 EUR depending on the type of technology installed (e.g. X-ray) (ii).
Textile recycling technologies: The costs of recycling textiles vary based on the recycling process used, ranging from 30-120 EUR/t output for mechanical recycling to 200-700 EUR/t output for chemical recycling. Operational expenses make up most of the recycling costs, with soft mechanical recycling costing up to 3,500 EUR/t output. Despite the high costs, soft mechanical recycling yields high-quality output and potential for greater revenue. Table 2 demonstrates a trade-off between cost and quality among available recycling technologies.
Indicative abatement cost: In the textile industry, it is estimated that it would cost around 130 EUR/ton CO2e to increase the end-of-use recycling by 15% (10). Paper recycling abatement costs are estimated to be lower, with some estimates reporting net savings due to recycling: -77 to 18 EUR/ tCO2e (11)(12)
Table 2: Estimated cost ranges of some textile recycling technologies at maturity
Technology | Investment required (EUR/t output) | Operational costs (EUR/t output) | Cost and quality trade-offs |
---|---|---|---|
Mechanical recycling | 30-90 | 200-800 | Mechanical recycling is among the most cost-competitive techniques, although fiber degradation may be a limiting challenge. |
Soft mechanical recycling | 330-360 | 2,800-3,500 | Although more expensive than traditional mechanical recycling, the higher quality output of this method presents greater revenue potential, which can offset the higher costs. |
Thermo-mechanical recycling | 100-120 | 400-850 | Cost competitive, although current use is limited due to strict feedstock purity requirements. |
Chemical recycling | 200-700 | 750-1,900 | Relatively expensive but benefiting from high-scale efficiencies and high-value fiber output. |
Source: McKinsey (13)
Potential co-benefits and side effects
Co-benefits
Environmental and health benefits from increased resource efficiency: Increased resource efficiency would enable to reduce pressure on the environment, from water resources to land-use. Additionally, recycling textile can reduce the need for chemicals that may have harmful effects on human health.
Employment benefits: Increasing sorting and recycling can have beneficial impact on employment, given that these processes would have to be largely scaled up and will create jobs for both the scale up of infrastructure and the process itself. Estimates found that around 15,000 new green jobs could be created in Europe by 2030 due to textile recycling (though impacts on employment in other regions should also be considered) (14).
Side-effects
Fiber quality deterioration: Many of the existing technologies for textile recycling are currently not able to prevent the deterioration of fibers. It is therefore necessary to complement recycled fibers with higher quality alternatives as a supplement for creating new yarn, increasing resources needs. Additionally, downcycling restricts the application of the fabric in clothing and textiles. Lower quality fibers might also lead to less durable products with a shorter lifespan. All these variables reduce the market and the value of recycled fibers and can create a competitive disadvantage. Nevertheless, the landscape is not devoid of remarkable technological progresses and successes, as start-ups and companies piloting innovative technologies are yielding encouraging outcomes (15).
Recycling trade-offs: environmental footprint: Recycling – paper or textiles – may not be the most environmentally sustainable option. Firstly, recycling, particularly for certain textiles and processes, can be very energy intensive, increasing emissions if the process is not powered by renewable energy. Additionally, large-scale textile recycling would require extensive chemical processing of mixed fiber fabrics, which can cause further GHG emissions and hazardous discharge to water systems. Given that dyeing textiles presents significant environmental challenges, the recycling process could therefore inadvertently create additional risks. Furthermore, the degradation of fibers requires the incorporation of new virgin fibers to produce new fabrics. However, recycling facilities (in developing countries) are often located at a significant distance from the textile manufacturing sites (in emerging economies). The transportation between these facilities results in substantial emissions, increasing the environmental footprint of textile recycling.
Textile recycling and green claims To minimize undesired trade-offs, it is important for brands to adequately emphasize the importance of reusing items before recycling, and implement the necessary measures to guarantee that recycling takes place. To satisfy a growing demand from consumers for recycled fibers, brands might be inclined to increase the share of recycled polyester from recycled plastic bottles, for example. This approach can inadvertently introduce readily recyclable plastic bottles into a supply chain where recycling technologies are still in the early stages of development, thereby reducing the emission abatement potential from plastic bottle recycling.
Implementation
These are the most common steps for paper manufacturers and textile producers to adopt sensor-based technologies and textile recycling.
Assess requirements: Conduct an assessment of existing recycling rates for products, recycling rates at owned facilities and use of recycled materials. Establish targets for the use of recyclable materials and corporate recycling rates, based on regulatory requirements, environmental targets and local infrastructure available to support recycling.
Evaluate potential to increase recycling rates and use of recycled materials: Identify new or existing products that could be manufactured either using materials that can be recycled for other purposes or produced directly using recycled materials, considering factors including product quality requirements and possibilities created by use of new sorting technologies. Additionally, identify opportunities to increase recycling of existing manufacturing waste.
Adapt existing infrastructure, processes and product design: Increasing recycling rates and use of recycled materials may require changes to the production process or modifications to existing machinery. Changes in product design can also reduce waste and increase the use of recycled materials.
Collaborate with recycling partners: Establish agreements with new partners sorting waste using sensor-based technology and encourage existing partners to adopt these technologies. Coordinate on a schedule for collection and transportation of: a) recycled materials to the manufacturing facility for reuse in the production process and b) the organization’s waste to be sorted and recycled by the recycling facility.
Key challenges/hurdles (16)
Varying sorting and collection rules: One of the most pressing challenges faced by multi-national organizations is the differences in the sorting and collection rules in different jurisdictions. If collection rules are not varied and unspecific about the separation of different types of waste, it is hard to develop a coordinated recycling strategy and more advanced and costly technologies may be required to actually sort the waste for recycling.
Inconsistencies in regulations around recycling and use of recycled inputs: This hinders the recycling process, as some regulations mandate the need to recycle but prohibit the use of recycled paper. The EU Commission has for instance adopted legislation restricting the type of input waste that can be used for food containers. The legislation also mandates the use of suitable technologies for recycling waste (17). This creates uncertainty for companies around which materials they can recycle for reuse.
Lack of infrastructure: Rules for sorting and collection also determine the available infrastructure in different jurisdictions. Less stringent collection rules often lead to a lack of appropriate infrastructure for sorting or collection.
Packaging and material design: Design of packaging of products (e.g. coating to preserve functionality of packaging) can make it challenging to recycle as the coating cannot always be removed.
Challenges in measurement of Scope 3 impact: Forest companies are disincentivized to invest in these solutions due to challenges inherent to scope 3 accounting.
Lack of scale in operations: Recycling facilities are generally large-scale projects requiring long-term investment. Critical scale across the value chain is required to provide sufficient feedstock for the necessary fiber-to-fiber recycling technologies to operate at scale. A lack of wide-spread adoption of recycling, may prevent recycling technologies operating at scale.
Potential solutions
Design for recycling: Manufacturers should follow a ‘design for recycling’ approach that helps them to prioritise materials which can be recycled following use. There are general guidelines available for different steps of manufacturing by various organizations, e.g. 4evergreen – a cross-industry alliance of over 100 members provides guidelines for fibre-based packaging (18).
Collaboration across the value chain: Addressing the key challenges in recycling necessitates a strong emphasis on collaboration between forestry companies, technology providers and recycling centres. The industry must also actively collaborate to establish ambitious recycling targets and diligently work towards achieving them, thereby ensuring the complete scalability of recycling operations. Coordinated support from forestry companies for advanced recycling technology can provide the necessary scale to establish the required infrastructure.
Engagement with public policymakers: Forestry companies can engage with governments to establish consistent regulation that encourages recycling, e.g. through restrictions on the export of unsorted waste and financial incentives to use recycled materials. Additionally, establishing national and industry level recycling targets and education programs can grow the circular economy.
Footnotes
(i) Note: Gemechu, E. D. et al. (2013). A comparison of the GHG emissions caused by manufacturing tissue paper from Virgin Pulp or Recycled Waste Paper;
(ii) Note: McKinsey analysis
Going further
Check out other Actions as part of the Forest Sector Net-Zero Roadmap:
Appendix
Methodology for assessment against criteria
Level of maturity: The level of maturity score for each action is based on the ‘Technology Readiness Level’ (TRL). This indicator estimates the maturity of technologies, measured through an assessment of their progress and capabilities. The scale originally ranges from 1 to 9, where TRL 1 is the lowest and TRL 9 is the highest. When a technology is at TRL 1, scientific research is underway and results are being translated into future research and development, while at TRL 9 the technology has already been proven to work. The International Energy Agency (IEA) has extended the TRL scale used in this report to incorporate two additional levels of readiness: one where the technology is commercial and competitive but needs further innovation efforts for the technology to be integrated into energy systems and value chains when deployed at scale (TRL 10). Finally, there is a level where the technology has achieved predictable growth (TRL 11).
Emission abatement potential: The emission abatement potential of each action, whether a technology or practice, describes the potential to reduce GHG emissions with respect to the counterfactual technology or practice, meaning the technology or practice that is part of the ‘business as usual’ scenario, or that is substituted or improved by adopting a decarbonization action. Emission abatement potential is usually expressed as a percentage, and the higher it is, the higher GHG emission reductions can be achieved:
Low = < 15% of GHG emissions Medium = 15-50% of GHG emissions High = > 50% of GHG emissions
Abatement cost: The abatement cost is an indicator that measures the costs associated with abating one ton of GHG emissions (EUR/ton CO2e abated). The lower the abatement cost, the cheaper it is to reduce emissions, and therefore the more attractive the action.
Short-term economic feasibility: Economic feasibility is assessed based on the maturity level and the abatement cost of each action, as per the visualization below. Actions with a low maturity level (≤ 4) are considered to have low economic feasibility in the short term, given the need to validate and deploy the technology in relevant environments. However, if the abatement cost has already proven to be low, the action is assessed as medium. Actions with medium maturity (TRL 5-8) may have low or medium economic feasibility in the short term, depending on the abatement cost (≤ 250 EUR/tCO2e). Similarly, the short-term economic feasibility of actions with higher maturity (TRL 9-11) varies depending on the abatement cost (low when < 550 EUR/tCO2e, medium if the cost ranges between 250-450 EUR/tCO2e, or high if the cost is lower than 250 EUR/tCO2e). The thresholds that determine short-term economic feasibility are defined by taking into consideration that additional benefit, including revenue generating opportunities. They are not monetized or included in the abatement cost estimates.