Increase carbon removals with sustainable forest management
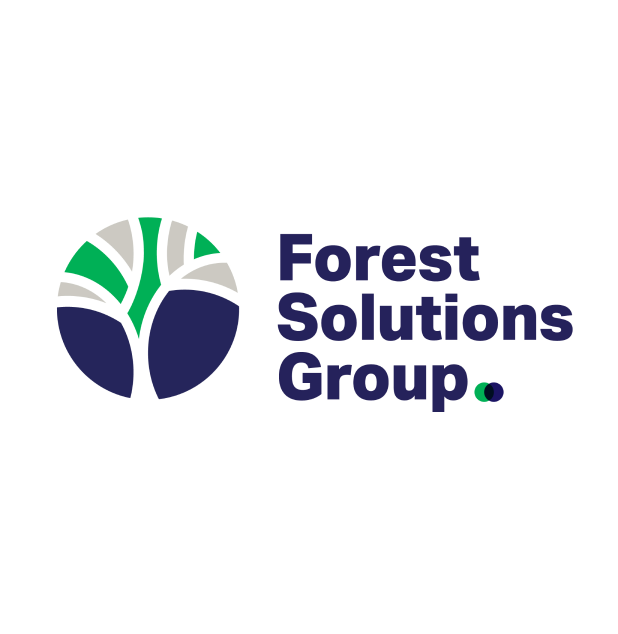
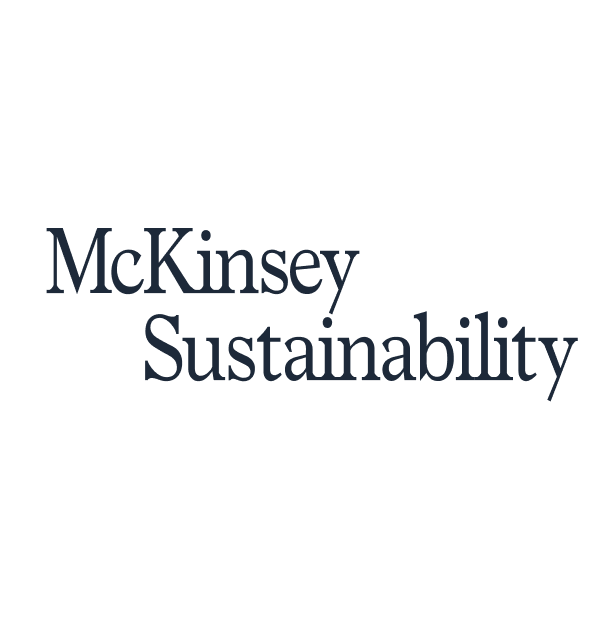
Summary
Sustainable forest management is critical for maintaining and increasing carbon removals in working forests, and reducing operational emissions associated with forest production
Context
This Action is one of ten from the Catalogue of Key Decarbonization Actions, part of the Forest Sector Net-Zero Roadmap. Its objective is to support forest companies in the choice and implementation of actions to address emission hotspots and leverage the greatest opportunities for carbon removals.
LEVEL OF MATURITY | EMISSION ABATEMENT POTENTIAL | SHORT-TERM ECONOMIC FEASIBILITY | |
---|---|---|---|
LOW TO HIGH | MEDIUM TO HIGH | LOW TO HIGH | |
• Carbon removals: Technologies available and widely adopted TRL 9-11 • Reduce emissions: Technologies partially available, not widely used TRL 4-6 | • Emission abatement potential varies depending on the technologies and practices adopted | • Low/medium economic feasibility for low maturity practices to reduce operational emissions, high economic feasibility for carbon removal practices |
See methodology in the appendix section
Solution
Forests are the largest terrestrial carbon sink, absorbing CO2 from the atmosphere and storing it for long periods of time in the trees and soil. Working forests transfer some of this carbon to forest products, where it is also stored for long periods of time. Sustainable forest management is key to securing these carbon stocks and ensuring these forests continue to sequester carbon. Forest managers and owners have a critical role to play in maintaining and increasing carbon removals in sustainable working forests, and reducing operational emissions associated with forest production.
As shown in Table 1, several practices can be implemented with the aim of:
Maintaining carbon stocks and removals: The most available practice is to maintain or enhance forest carbon stocks by ensuring growth is equal to or greater than the combination of harvesting and mortality. Practices in this space are aimed at preventing the loss of carbon by mitigating natural disturbances, such as pests and disease, and avoiding major carbon losses from forest fires. This includes monitoring for fire and pest outbreaks to provide early warnings and coordinate the response to minimize damage. In addition to conventional methods like ground-based monitoring, innovative technologies such as unmanned aerial vehicles (i.e., drones), remote sensing and weather monitoring can support forest managers in monitoring forests. In addition, climate resilience – that is, the capacity of forests to cope with hazardous events or disturbances due to climate change – could be enhanced through prescribed fires in ecologies where this measure is appropriate and supportive of biodiversity outcomes. This can reduce the amount of leaf litter, pine needles, shrubs and other fuels in the forest, thus reducing the risk of wildfires. Other measures for building climate resilience in managed forests include enhancing road infrastructure to ensure forest access (thus aiding fire response), changing the timing of harvesting to accommodate altered timing of spring thaws, and monitoring and management for disease and pests (1) (2). In addition to the critical role of forest conservation and restoration, the IPCC emphasizes the critical role that sustainable forest management plays in maintaining and enhancing carbon stocks in working forests as forest degradation and conversion of forests to other land uses are major drivers of carbon release in the atmosphere (3).
Enhancing carbon removals: The amount of carbon dioxide sequestered from the atmosphere can be increased by optimizing management and harvesting practices. Such practices should be tailored for the regional and subregional diversity of forestry ecologies (both domestically and globally). Examples of practices that could increase carbon sequestration rates include pruning and thinning, introducing species or genotypes that are better adapted to future conditions, changing the forest structure and targeted fertilization (4).
Reducing operational emissions: Forest managers can also take action to reduce GHG emissions from forestry operations such as reducing fuel use in harvesting equipment, replacing fuel with lower-emitting fuels, and/or moving to electric equipment, as appropriate. Additionally, reducing the amount of fertilizer used, or ensuring it is only used where necessary and will be absorbed by the growing trees. It is important, however, not to reduce the net impact of the forests’ removal capability with decreased fertilizer application.
Table 1: Non-exhaustive list of technologies and practices to reduce operational emissions and maintain and increase carbon removals
★ Reduce operational GHG emissions ▲ Maintain carbon removals ⬤ Increase carbon removals
Practice/technology | Description | Impact |
---|---|---|
Adoption of low-emission machinery | The forest sector can improve fuel efficiency and reduce emissions by adopting hybrid or electric machinery and vehicles (e.g. electric or hybrid forwarders, harvesters and skidders), as well as machinery powered by hydrogen and biomass-based fuels, which are currently under development. In addition, companies can save fuel by optimizing transportation routes and considering multimodal transportation opportunities combining freight via road and rail (5). | ★ |
Reduce wildfire risk and damage | In some ecologies, prescribed burning is used as a preventive measure to reduce wildfire risks and preserve forests (helping to reduce emissions (6)). Forest managers can complement this approach with additional practices to lower both the frequency and intensity of wildfires, such as by clearing small trees from dense areas, reducing forestry slashing, thinning trees or increasing spacing between trees. In addition, recently developed forest fire detection systems based on artificial intelligence are instrumental for detecting and localizing forest fires at an early stage to minimize damage (7). Once a wildfire has been detected, such systems can also be leveraged to predict the spread of a fire, which will benefit incident commanders to make critical judgment calls, such as where to send their limited firefighting personnel. Aerial firefighting through drones constitute another effective but costly measure for minimizing the damage of wildfires, as in contrast to conventional human-piloted firefighting aircraft, they can fly after dark and in smoky conditions. | ★ ▲ |
Optimize fertilizer application, and adopt inhibitors | The adoption of best management practices for fertilization is vital to both reducing emissions and increasing carbon removals. Supplemental nutrient applications can increase forest carbon sequestration capacity by both fostering plant growth and increasing litterfall and deposition of organic material on the soil. However, application of fertilizer to increase carbon removals must be targeted to the specific site, and optimized in terms of frequency, amount and rate of fertilization in order to increase nitrogen use efficiency. In fact, maximizing the share of nutrients taken up by the trees reduces nutrient losses to the environment, preventing potential detrimental impacts on air quality and on water resources (8). Targeted fertilization can be supported by digital technologies to inform site-specific management. Additionally, increasing the adoption of inhibitors and controlled-release fertilizers can further reduce GHG emissions. | ★ ⬤
|
Pruning, thinning and partial cutting | • In some ecologies, pruning improves stem quality, while thinning reduces competition for light, water, and nutrients, thereby improving tree growth, biomass and soil carbon content. Similarly, partial cutting – that is, harvesting only a subset of trees from a forest to select high quality trees – was found to have a positive impact on carbon content in soil (9). | ★ ▲ ⬤ |
Optimizing harvest rotation lengths | Rotation length can impact the rate at which carbon is sequestered by growing trees and forests. Optimizing this process depends on the tree species, climatic zone and harvesting goals. | ▲ ⬤ |
Incorporating mixed species into plantations | Incorporating different species into forest plantations can help to improve resilience to droughts, pests, diseases and wildfires as well as increase the carbon sequestration potential of a stand (10). Although a few studies highlight potential productivity gains from mixed species production models (11) (12), their viability largely depends on the existence of profitable markets for the grown timber. | ▲ ⬤ |
Tree genetics | Breeding of fast growing tree species well adapted to the location and resilient to the effects of climate change contributes to maintaining and enhancing carbon sequestration, while increasing productivity (13)(14). | ▲ ⬤ |
Use of technology | The use of digital data capture and planning contributes to the implementation of more targeted forest management practices and tight operational control. Some example practices involve: site-specific fertilization treatment based on granular assessment of soil nutrient deficiencies, digital forest inventory using drones and lidar, fully mechanized harvesting integrated with supply chain planning, satellites and drones to provide early fire detection. | ★ ▲ ⬤ |
Usage
The adoption of practices and technologies to reduce operational emissions, as well as to maintain and increase carbon removals, largely varies depending on the type of action: technologies to reduce operational emissions, including precision forestry, are largely at a prototype stage and therefore adoption is limited (TRL 3-5). However, sustainable forestry practices to maintain and enhance carbon removals – such as thinning and pruning, are often used, particularly for larger scale forest managers (TRL 9-11).
Table 2: Adoption of technologies/practices to reduce operational emissions, and maintain or increase carbon removals
Impact | Technology / practices | Level of maturity (TRL 1 - 11) | Current adoption | Barriers to adoption |
---|---|---|---|---|
Reduce operational emissions | Adoption of low-emission machinery | Low maturity (TRL 4/5) | Electric machinery for forest operations is currently being developed, especially forwarders, harvesters and skidders, although most projects are still in a pilot phase (15). Hydrogen fuel cells are under development for the agricultural sector (in tractors) and may become available for the forest sector, although the technology still requires refinement and further development before adaptation at full scale. | Electrification of machinery and vehicles within forestry faces unique challenges compared to automotive applications. For instance, forestry machinery operates in rural or off-grid areas where there is limited access to charging infrastructure, meaning batteries must last long enough to cover for limited charging infrastructure (16). |
Optimize fertilizer application, and adopt inhibitors | Medium maturity (TRL 6) | Fertilizer inhibitors also require further research and product development to make these technologies more affordable, better understand the synergies between them, and improve understanding of wider environmental impacts (17). | Low maturity, high costs, variability in effects, which is highly dependent on soil chemistry, climate and nutrient availability, regulatory constraints. | |
Maintain or increase carbon removals | Innovative technologies for precision forestry | High maturity (TRL 9-11) | Technologies to support precision forestry, such as drones and software already exist and are commercially available. However, precision forestry techniques are still limited to a few players (18). | High initial investment costs, uncertain returns and results, low institutional support and technological complexity (i). |
Other sustainable forestry practices to maintain and increase carbon removals | High maturity (TRL 11) | Conventional sustainable forestry practices, such as pruning, thinning, practices for sustainable intensification (e.g. tree genetics and seedling selection) are all widely adopted in the forest sector. | Costs and uncertainty as to the financial benefits, inadequate regulatory and market incentives, lack of information and guidance. |
Impact
Climate impact
Targeted emissions sources
The adoption of technologies and best practices to reduce operational emissions targets GHG emissions from, for example, vehicle fuels, fertilizer volatilization, and others, whereas practices and technologies to maintain and increase carbon removals are negative-emission solutions aimed at storing carbon in biomass, soils, and forest products.
Emission abatement potential
The emission abatement potential of sustainable forest management to reduce operational emissions will largely vary based on the type of practices adopted: improved energy efficiency due to hybrid propulsion systems in forest vehicles would result in a significant reduction in GHG emissions, depending on the type of vehicle (hybrid or fully electric) and the energy mix from the grid. Inhibitor technologies can achieve up to 50% emission reduction compared to no use (19).
The impact of sustainable management practices to maintain and increase carbon removals will also largely vary based on the type of practice and the specific context. In an Australian case study, pruning was found to improve carbon sequestration potential by 33% and 62% six weeks after upper and middle crown treatments, with a significant effect lasting for 40 weeks (20). Choosing tree species with strong carbon sequestration properties may also be effective. For example, Scot pines and the soil beneath them were found to sequester around 33% less carbon than oak trees in another case study (21).
Business impact
Benefits
Reduce operating costs: Some technologies relevant to reducing emissions and maintaining carbon removals also reduce costs. These include forest-mapping drones (cost effective compared to ground methods) and targeted fertilizer application. Other examples are electric tractors that cost less to recharge than fuel-powered alternatives or management software. For instance, wood logistic optimization software can lower delivery costs of wood to mills by 2.5% (22).
Increased revenue by boosting productivity and securing forest assets: Increased productivity not only increases carbon removals but also potentially generates additional revenue by providing more timber (23). By improving forest resilience to climate change, forest managers are securing forest assets for the future, thereby reassuring investors and regulators, which can facilitate access and reduce capital costs.
In some scenarios, carbon credit payments for increasing carbon removals may cause a forest manager to optimize the land for carbon, in addition to or instead of timber. Forest carbon credits would need to meet integrity requirements such as additionality, permanence and leakage.
Costs
Investment required: As for electric machineries, estimates project electric (yard) tractors will cost around 160,000-275,000 EUR (compared to about 115,000 EUR for a typical diesel tractor) (24)
Operating costs: Some recurring spending will also be required. For instance, nitrification inhibitors could cost 24 EUR/ha, GPS surveying is estimated to cost 90 EUR/ha (25), while some software for regenerative forestry cost about 770 EUR/year (ii)
Indicative abatement cost: Because of the wide range of technologies that can be used in sustainable forest management, abatement costs may vary substantially. It is estimated that many forestry mitigation options (including sustainable forest management) could help lower emissions for about 20 EUR/tCO2 (25). Some technologies may even have negative abatement cost due to co-benefits. For instance, the abatement cost of nitrification inhibitors is estimated to range from 14-34 EUR/tCO2e due to higher expected yield and a reduced impact on nature, while electrification of machinery could lead to net savings of -66EUR/tCO2e due to lower fuel costs.
Potential co-benefits and side effects
Co-benefits
Biodiversity protection: Some practices, such as increased species diversity, can have a beneficial impact on biodiversity. Benefits can be high in areas with many endemic species or in regions that used to be prone to deforestation (26). Investment in research and involvement of experts will help prevent potential detrimental impacts in specific ecologies.
Air quality: Reducing the frequency and intensity of wildfires will benefits local air quality and health outcomes as wildfires are known to release a range of harmful air pollutants, including cancer-causing substances to tiny particles that can increase the risk of a heart attack or stroke.
Soil health: Practices that maintain and increase carbon removals by increasing soil organic carbon also directly impact soil health. In turn, this may have positive impacts on climate adaptation, by preventing soil erosion and flood damage. This is expected to become increasingly important in the future, as forests could help adapt against the projected increase in extreme precipitation events (27).
Water quality: Sustainable forest management can improve water quality due to better retention of nitrogen and phosphorus by the vegetation due to better forest health, as well as fewer instances of over applying fertilizers (28).
Side-effects
Site-specific assessments: Sustainable forest management practices need site-specific assessments, which may require significant human resources and time.
Increased reliance on the grid: Machines need to have a long autonomy as there are likely no charging capacities in the forest. This is particularly important for vehicles such as tractors, in order to avoid operators having to recharge vehicles too often or risk being stuck in an area difficult to access.
Implementation
These are the most common steps to implement measures to enhance carbon removals and reduce emissions in sustainable working forests:
Manage the forests that are owned, leased or managed to the highest standards of sustainable forest management: Organizations should follow guidelines prescribed by globally recognized forest certification systems such as Forest Stewardship Council (FSC), Programme for Endorsement of Forest Certification (PEFC) and Sustainable Forestry Initiative (SFI).
Identify appropriate actions to implement: The organization should assess the different technologies/practices that can be applied within the operations. This includes an assessment of their relevance based on the operation and characteristics of the forests (e.g. tree species, location), and of their economic feasibility. It is important to note that the size and level of maturity of the company can potentially be a limiting factor in the implementation of some of these actions.
Engage with forest managers: Companies that do not own forests should engage with suppliers and forest managers to uphold high standards of forest management and to encourage the use of technologies to remove carbon and reduce emissions.
Certify efforts to increase removals: Reputable and high-integrity carbon creditcertification programs for carbon removals and capture schemes (e.g. Verra, Gold Standard) demonstrate the company’s adherence to rigorous standards and best practice in environmental sustainability. In addition to being a pre-requisite to sell credits in voluntary and compliance carbon markets, they also serve as powerful tools to demonstrate transparency and accountability in decarbonization efforts.
Key challenges/hurdles
Accurate measurement of carbon sequestration: In many regions there is a lack of affordable carbon measurement services and expertise for use by forest companies. In addition, in some cases, unclear rules and guidelines around carbon sequestration and mapping of land systems can create uncertainty around mitigation measures and deter investment in abatement mechanisms. When organizations do not own the forests that they manage, this can further increase the challenge in implementing these measures in the absence of the right incentives for forest owners to implement them.
High costs and low margins from forest management: The investment required for emissions reduction technologies come in addition to already high costs of forest management, exacerbated by rising land prices. Moreover, due to concerns around the legitimacy of claims, extracting high premiums from improved forest management practices is difficult, and margins are low as a result. The additional costs from emissions reduction technologies, however, may be partly offset by increased productivity and the benefits from the improved forest resilience to the effects of climate change.
Potential solutions
Building a highly skilled workforce in forest management: Organizations should focus on building the right skills in their workforce to lead actions on climate change mitigation.
Engagement with policymakers on carbon sequestration data: Organizations may work with policymakers to improve access to better quality forest carbon data and decision-making tools, as well as on improving governance mechanisms to determine land ownership and ensure the protection of human rights.
Invest in R&D: Organizations can invest in cooperative research programs to lower cost and facilitate the adoption of mitigation technologies (e.g. fertilizer inhibitors and low emissions-machinery), improved forest management practices, mapping and measurement of carbon emissions from initiatives.
Footnotes
(i) Note: Long, T.B. et al. (2015). Barriers to the adoption and diffusion of technological innovations for climate-smart agriculture in Europe: evidence from the Netherlands, France, Switzerland and Italy.
(ii) Note: Dyck, B (2003). Precision forestry—The path to increased profitability. Proceedings of the Second International Precision Forestry Symposium.
Going further
Check out other Actions as part of the Forest Sector Net-Zero Roadmap:
Appendix
Methodology for assessment against criteria
Level of maturity: The level of maturity score for each action is based on the ‘Technology Readiness Level’ (TRL). This indicator estimates the maturity of technologies, measured through an assessment of their progress and capabilities. The scale originally ranges from 1 to 9, where TRL 1 is the lowest and TRL 9 is the highest. When a technology is at TRL 1, scientific research is underway and results are being translated into future research and development, while at TRL 9 the technology has already been proven to work. The International Energy Agency (IEA) has extended the TRL scale used in this report to incorporate two additional levels of readiness: one where the technology is commercial and competitive but needs further innovation efforts for the technology to be integrated into energy systems and value chains when deployed at scale (TRL 10). Finally, there is a level where the technology has achieved predictable growth (TRL 11).
Emission abatement potential: The emission abatement potential of each action, whether a technology or practice, describes the potential to reduce GHG emissions with respect to the counterfactual technology or practice, meaning the technology or practice that is part of the ‘business as usual’ scenario, or that is substituted or improved by adopting a decarbonization action. Emission abatement potential is usually expressed as a percentage, and the higher it is, the higher GHG emission reductions can be achieved:
Low = < 15% of GHG emissions Medium = 15-50% of GHG emissions High = > 50% of GHG emissions
Abatement cost: The abatement cost is an indicator that measures the costs associated with abating one ton of GHG emissions (EUR/ton CO2e abated). The lower the abatement cost, the cheaper it is to reduce emissions, and therefore the more attractive the action.
Short-term economic feasibility: Economic feasibility is assessed based on the maturity level and the abatement cost of each action, as per the visualization below. Actions with a low maturity level (≤ 4) are considered to have low economic feasibility in the short term, given the need to validate and deploy the technology in relevant environments. However, if the abatement cost has already proven to be low, the action is assessed as medium. Actions with medium maturity (TRL 5-8) may have low or medium economic feasibility in the short term, depending on the abatement cost (≤ 250 EUR/tCO2e). Similarly, the short-term economic feasibility of actions with higher maturity (TRL 9-11) varies depending on the abatement cost (low when < 550 EUR/tCO2e, medium if the cost ranges between 250-450 EUR/tCO2e, or high if the cost is lower than 250 EUR/tCO2e). The thresholds that determine short-term economic feasibility are defined by taking into consideration that additional benefit, including revenue generating opportunities. They are not monetized or included in the abatement cost estimates.